General Chemical Properties of Non Metals
The non-metals are very reactive and most of them react with other elements to form different compounds.
The following are important chemical properties of non-metals which are connected with their tendency towards electron gain in the course of formation of compounds:
1. The oxide of a non-metal is either acidic or neutral but never basic. The oxide of a non-metal is a covalent compound. Being acidic, it combines with water to form an acid, e.g.
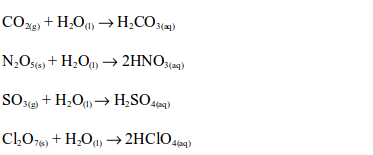
2. A non-metal never replaces hydrogen in an acid to form a salt. This is because replacement of hydrogen in an acid is due to the fact that H+ accepts electrons supplied by a metallic atom.

A non-metal is an electron acceptor and so cannot release electrons to hydrogen ions in solution.
3. Non-metals form covalent chlorides, for example, the behaviour of phosphorus forming its chlorides is well known.

A covalent chloride like this is usually a volatile liquid, a non electrolyte, and rapidly hydrolysed by water.

These properties are characteristic of non metallic chlorides (except CCl4 which is not hydrolysed by water).
4. Non-metals combine with hydrogen to form many hydrides. A covalent compound is formed by equal sharing of electrons between or among the combining atoms. For example, methane ammonia, hydrogen chloride and hydrogen sulphide are the covalent compounds.
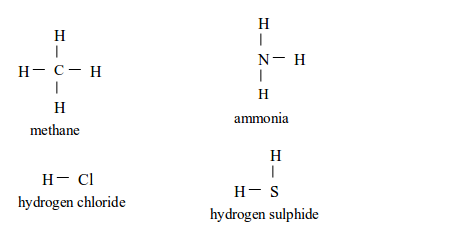
5. Non-metals are oxidizing agents As discussed early, non-metals accept electrons from other substances. Therefore, they are called oxidizing agents because, upon accepting the electrons, the substances donating these electrons are oxidized. So they act as the agents for oxidation of other substances.
The Oxidizing Properties of Non-metals
Explain the oxidizing properties of non-metals
Non-metals react by gaining electrons to become negative ions. A univalent non-metal accepts one electron while a divalent one accepts two electrons. The ion formed carries the corresponding number of negative charges, but they rarely exceed two and never exceed three.

When a substance loses electron(s), it becomes oxidized, i.e. its oxidation number increases. This is called oxidation. Due to the fact that non-metals accept the electrons(s) donated by other substances, particularly metals, they are, therefore, termed as oxidizing agents. This is because by accepting the electrons, they help oxidize the electron donors.
Those substances or metals which donated the electrons are called reducing agents. This is because the electrons they donate reduce the oxidation number of non-metals. This process is called reduction. In this respect, non-metals act as oxidizing agents while metals act as reducing agents.
Strong and weak oxidants
As we have already seen, non-metals ionize by electron gain. In all cases, the extra electron(s) accepted lead to the formation of negative ions. The easiness of formation of negative ions depends on the ability of an element to accept the electrons. The ability of accepting electrons is called electronegativity of an element. Some elements are more electronegative than others.
The order of electronegativity of some non-metals is as follows: Fluorine < Chlorine > Bromine > Iodine > Nitrogen > Carbon
The degree of electronegativity indicates reactivity and hence oxidizing power of the element. Elements with higher electronegativity will displace those elements with lower electronegativity from their compounds.
Referring to the series above, fluorine will displace all the rest of the elements from their compounds as it is more electronegative than any other element in the series. Likewise, chlorine can displace bromine, iodine and nitrogen from their compounds. The displacement reaction occurs in the manner:

Where X is more electronegative than Y
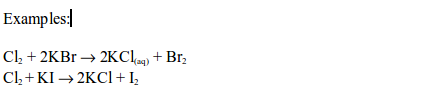
The higher the electronegativity the stronger the oxidant. For example, bromine is a stronger oxidant than iodine, nitrogen and carbon.
The Displacement of Non-metals by another Non-metal from a Compound
Describe the displacement of non-metals by another non-metal from a compound
Non-metals in the reactivity series
It is useful to placecarbonandhydrogeninto the reactivity series because these elements can be used to extract metals.
Here is the reactivity series including carbon and hydrogen:
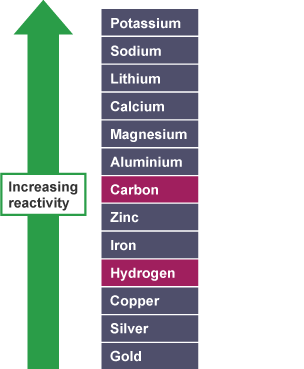
Note that zinc and iron can bedisplacedfrom theiroxidesusing carbon but not using hydrogen. However, copper can be extracted using carbon or hydrogen.
Chlorine
Chlorine is very reactive, so it is never found as the free element in nature. It occurs mainly as sodium chloride or rock salt. It also occurs in the combined state as chlorides of sodium, potassium and magnesium.
How is chlorine made?
In industry, chlorine is made by electrolysis of molten sodium chloride or brine. Brine is a concentrated solution of sodium chloride in water.
In the laboratory, chlorine is made by the oxidation of concentrated hydrochloric acid. The oxidation can be brought about by a number of oxidizing agents, for example, lead (IV) oxide, manganese (IV) oxide, trilead tetraoxide (Pb3O4) or potassium manganate (VII).
The reaction equation is:

When potassium permanganate is used, reaction equation is:

The poisonous nature of chlorine
Chlorine is a useful but dangerous gas. It is very poisonous if inhaled to even a small extent (1 part of chlorine in 50,000 parts of air causes death). Chlorine poisoning occurs when the gas is inhaled or swallowed. It reacts with water inside and outside of the body (such as water in the digestive tract and moisture on the lungs and eyes) to form hydrochloric acid and hydrochlorous acid. Both of these substances are extremely poisonous.
Most incidents of chlorine poisoning are due to ingesting household cleaners or bleach products. Due to its poisonous nature, chlorine was used in the World War I (1914-1918) to kill people and it caused many deaths.
The Chemical Properties of Chlorine
Describe the chemical properties of chlorine
1. When a little litmus solution is poured onto a gas jar of chlorine, litmus immediately turns colourless. The gas also bleaches a damp litmus paper since the gas is acidic. If blue litmus paper is used, it is first turned red before being bleached. The bleaching action is due to the fact that chlorine reacts with the water, forming a mixture of hypochlorous and hydrochloric acids.

The hypochlorous acid is a very reactive compound and readily gives up its oxygen to the dye, to form a colourless compound:

Thus, dry chlorine does not bleach. It has to be moist since the hypochlorous acid formed by its reaction with water is the one that is responsible for the bleaching action.
2. Chlorine reacts with many reactive metals, on heating, giving their respective chlorine salts, e.g.:
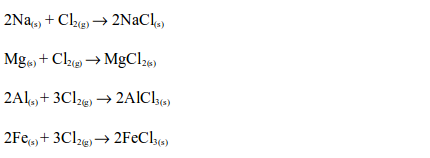
3. Some non-metals burn in chlorine to form covalent compounds:
(i) Dry, yellow phosphorous burns spontaneously in the gas to produce white fumes of chlorides of phosphorous, mainly PCl3.
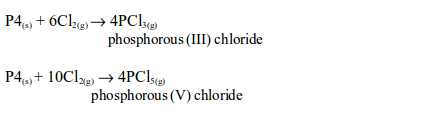
4. When a filter paper dipped into a little turpentine is dropped into a gas jar of chlorine, a violent reaction occurs and a black cloud of solid particles of carbon is formed.

The reaction also takes place with other hydrocarbons as well and as with turpentine, hydrogen chloride gas is formed. The hydrogen chloride can be shown to be present by passing a little ammonia gas across the top of the jar whereby dense white fumes of ammonium chloride are observed.

5. Hydrogen chloride gas is oxidized to elemental sulphur by chlorine gas. When a gas jar of hydrogen sulphide is inverted over a gas jar of chlorine, a yellow precipitate of sulphur and hydrogen chloride gas will be formed.

6. When a stream of chlorine gas is bubbled through a pale green iron (II) chloride solution, a red-brown precipitate of iron (III) chloride is formed, showing that the iron (II) chloride has been oxidized to iron (III) chloride.

Ionically:

7. When chlorine is bubbled into the solution of sulphur dioxide in water for a few minutes, dilute sulphuric acid is obtained.

8. The reaction between chlorine and alkalis gives products which depend upon reaction conditions:
Chlorine reacts with cold dilute aqueous solution of sodium or potassium hydroxides, forming a pale yellow solution of the hypochlorite and chloride of the metal.

With the hot concentrated aqueous solution, a mixture of the chloride and chlorate is formed.

A similar reaction occurs if hot concentrated calcium hydroxide solution is used.
The Uses of Chlorine
Explain the uses of chlorine
1. Chlorine is bleaching agent and is also used in the manufacture of other bleaches. When chlorine is added to sodium hydroxide solution, bleach is made.

The active chemical in bleach is sodium hypochlorite (sodium chlorate (I))
If chlorine is passed for a considerable time over solid calcium hydroxide the product formed is bleaching powder.

Bleaching power finds extensive use in dye works, and in laundries. It is used in industries where cloth, cotton, paper, etc. need to be bleached. Many textile industries use chlorine for bleaching purposes. Bleach is also used to kill bacteria for example in the toilet. It will also remove colour from the dyed materials.
2. Chlorine is added to water supplied to homes and industries to kill disease-causing germs like bacteria. If they were not killed, these pathogens might cause diseases such as cholera and typhoid. It is also used to sterilize the water in swimming pools.
3. Chlorine is used to make some important chemicals such as hydrochloric acid, chlorofluorocarbons (CFCs), tetrachloromethane (CCl4), and chloroform (CHCl3).
CFCs are carbon compounds containing both chlorine and fluorine. An example is trichchlorofluoromethane (CCl3F).
CFCs are very uncreative compounds. They were used in aerosol cans and refrigerators. However, CFCs damage the ozone layer. Consequently, their use is strongly discouraged.
4. Chlorine is a reactant in the manufacture organo-chloro compounds which are used to make pesticides, antiseptics (e.g. dettol), herbicides (weed killers) and polyvinyl chloride (PVC) which is an intermediate compound in the production of plastics.
Hydrogen Chloride
A Dry Sample of Hydrogen Chloride Gas
Prepare a dry sample of hydrogen chloride gas
Hydrogen chloride is a gaseous compound at room temperature. It is usually prepared in the laboratory by the reaction between concentrated sulphuric acid and any chloride, e.g. sodium chloride. When a mixture of the two is gently warmed, hydrogen chloride gas is formed.
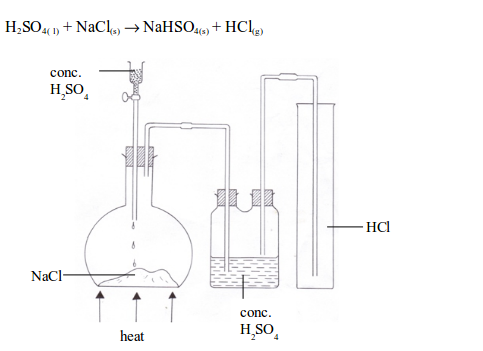
Preparation of hydrogen chloride gas
The Properties of Hydrogen Chloride Gas
Explain the properties of hydrogen chloride gas
(a) Physical properties
Includes
- It is a colourless gas with a choking, irritating smell and an acid taste.
- It is heavier (denser) than air.
- It fumes in most air due to the formation of tiny droplets of hydrochloric acid.
- It is very soluble in water (450 cm3 of gas in 1 cm3 of water). The acidic properties and the solubility of hydrogen chloride gas can best be shown by the fountain experiment
b) Chemical properties
1.A dry sample of hydrogen chloride gas has no effect on dry, blue litmus paper but it turns moist, blue litmus paper to red. This is due to acidic properties of hydrogen chlorine gas.
2. It does not burn and does not support combustion.
3. When a stream of hydrogen chloride gas is passed over some heated metals, the anhydrous chloride of these metals are formed:
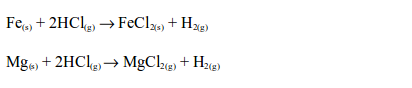
4. It is decomposed by strong oxidizing agents such as manganese dioxide, lead dioxide and red lead to give chlorine gas.
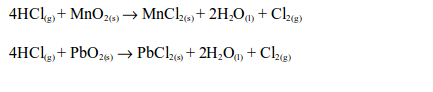
5. When the gas is passed though a solution of silver nitrate, acidified with dilute nitric acid, a white precipitate of silver chloride is formed. This is another test for hydrogen chloride gas and all soluble chlorides.

6. It gives clouds of white fumes when brought into contact with ammonia vapour. The fumes are solid ammonium chloride (NH4Cl).

In qualitative analysis, this is the chemical test for hydrogen chloride gas as well as for ammonia gas.
Reactions of aqueous hydrogen chloride
Hydrogen chloride gas is very soluble in water (and in other polar solvents). In water, an acid solution is formed, which is hydrochloric acid. In aqueous solution, the hydrogen chloride molecule dissociates into hydrogen ions (H+) and chloride ions (Cl-):

The solution is called hydrochloric acid. Hydrochloric acid reacts with metals, metal oxides, hydroxides (soluble bases) and metal carbonates..
This suggests that when hydrogen chloride gas is dissolved in water an acidic solution is formed, for example:
- it turns damp blue litmus paper red;
- it reacts with various substances just like other acids (see table bellow); and
- it conducts electricity, yielding hydrogen gas at the cathode and chlorine gas at the anode.
Reactions of aqueous hydrogen chloride
Acid reacting with | General equation |
oxide (base) | acid + metal oxide ® salt + water |
alkali (soluble base) | acid+metal hydroxide(akali)®salt+water |
metal | acid + metal ® salt + hydrogen |
metal carbonate | acid + metal carbonate®salt+water+CO2 |
The Uses of Hydrogen Chloride
Explain the uses of hydrogen chloride
These are:
- It is chiefly used in the production of hydrochloric acid. When the gas is dissolved in water in the appropriate proportions, hydrochloric acid is formed.
- Aqueous hydrogen chloride is used in qualitative and quantitative analysis.
- It is an important reagent in other industrial chemical transformations, e.g. hydrochlorination of rubber and production of vinyl and alkyl chlorides.
- In the electronics industry, it is used to both rub semiconductor crystals and to purify silicon.
- It is used in the textile industry, to separate cotton from wool and fluff.
- In the laboratory, anhydrous forms of the gas are particularly useful for generating chloride-based Lewis acids.
- It is used to remove rust from the oxidized metals.
- It is extensively used in the manufacture of medicines and is a key substance utilized to turn maize and other agricultural products into artificial sweeteners.
Sulphur
Occurrence
Sulphur is a yellow, crystalline, non-metallic solid. Its symbol is S. It has an atomic number of 16. Sulphur exists in nature as a free element and in compounds.
As a free element, sulphur is found in several countries such as Italy, Mexico, Japan, Poland, USA and Sicily. In its combined state, sulphur is found combined with metal ores such as galena (PbS), iron pyrites (FeS2), Copper pyrites (CuFeS2) and zinc blend (ZnS). It is also found in natural gas as hydrogen sulphide (H2S) and in crude oil as organic sulphur compounds.
The Extraction of Sulphur from Natural Deposits
Describe the extraction of sulphur from natural deposits
Sulphur is extracted from its underground deposits by the Frasch process. The Frasch process makes use of the low melting point (119oC) of sulphur.
In this process, a hole about 30 cm in diameter is bored down through the clay, sand, and limestone to the sulphur beds. This boring is lined with an iron pipe and inside the pipe, is sunk a device called sulphur pump. The pump consists of three concentric pipes (cylindrical pipes with a common centre) which end in a reservoir of a large diameter (see figure bellow).
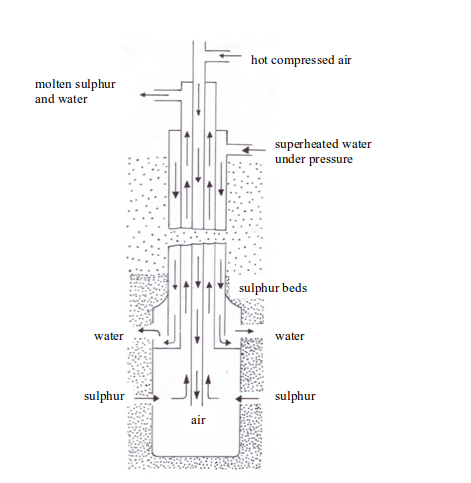
Extraction of sulphur
Down the outermost of the three pipes is forced a stream of water at about 170oC. This water must be kept at a pressure of about 10 atmospheres to maintain it in the liquid state, i.e. it is superheated water, and it is hot enough to melt the sulphur. The molten sulphur flows into the reservoir at the base of the pump and is forced up to the surface. The sulphur obtained is 99.5% pure and can be used without any purification.
The stages involved in the entire extraction process can be summarized as follows:
- Superheated water (170oC) is pumped through the outer pipe to melt the sulphur.
- Hot compressed air (10 atm) is pumped down through the inner pipe. The combination of the hot water and the hot air melts the sulphur. The molten sulphur, hot air and hot water form a froth.
- The froth is forced to the earth’s surface through the middle pipe by the compressed air.
- The molten sulphur is collected in large tanks (where the water drains off), cooled and solidified.
The Properties of Sulphur
Explain the properties of sulphur
Sulphur is a relatively reactive element that readily reacts with other elements to form compounds such as oxides, chlorides and sulphides.
In chemical reactions, sulphur exhibits both oxidizing and reducing properties.
1. Oxidizing properties of sulphur
Heated sulphur reacts with metals such as iron, copper, zinc and tin to give metal sulphides.
When a mixture of iron filings and powdered sulphur, in the proportion of 56 to 32, that is 7:4 (the ratio of their relative atomic masses), is heated, the two react in a highly exothermic reaction. The heat given out makes the mixture to continue glowing even after the heating has stopped. A black or dark grey iron (II) sulphide is formed.

In this reaction, sulphur acts as an oxidizing agent which oxidizes iron metal to iron (II) ion.

Sulphur is reduced to sulphur ion

Iron (II) sulphide is not attracted by a magnet since it is not magnetic. The magnetic property of iron is lost when this compound is formed.
The copper powder reacts with sulphur in the same way to form copper (II) sulphide.

2. Reducing properties of sulphur
Dilute acids do not act upon sulphur. However, sulphur is oxidized by hot concentrated sulphuric acid with the formation of sulphur dioxide.

In this reaction, the sulphur is oxidized by the acid to sulphur dioxide and the acid is reduced to the same substance.
The filter paper soaked in potassium dichromate changes from orange to green. The sulphur dioxide reduces chromium from chromium (VI) to chromium (III).

When sulphur reacts with hot concentrated nitric acid, brown fumes of nitrogen dioxide are formed.

Sulphur is oxidized by hot concentrates nitric acid to sulphuric acid while nitric acid is reduced to nitrogen dioxide and water.
The Uses of Sulphur
Explain the uses of sulphur
Includes
- Most of the sulphur produced in the world (90%) is used to manufacture sulphuric acid. Sulphuric acid is an important reagent in many industrial processes.
- Sulphur is used in the manufacture of sulphur dioxide (used in the Contact Process for the manufacture of sulphuric acid).
- Manufacture of calcium hydrogensulphite, Ca(HSO3)2, and sodium sulphite which are used for bleaching wood straw and wood pulp in the paper industry.
- It is also used for vulcanization of natural rubber. Rubber is usually sticky and soft. When heated with sulphur (vulcanization), it becomes hard and strong.
- It is used for dusting vines to prevent growth of certain kinds of fungi and also as an insecticide.
- Sulphur is used in smaller quantities for the manufacture of dyes, explosives, fireworks, gunpowder etc. For example gunpowder is a mixture of potassium nitrate, carbon and sulphur.
- It is used in the manufacture of various organic compounds such as plastics and pharmaceuticals like sulphur ointments e.g. sulphadimadine, septrin e.tc.
- Photographic chemicals such as carbon disulphide (CS2) and sodium thiosulphate (Na2S2O3) are made using sulphur as one of the raw materials.
- Some is added to cement to make sulphur concrete. Unlike ordinary cement, this is not attacked by the acid. So it is used for walls and floors in plants where acid is used.
Sulphur Dioxide
Preparation of sulphur dioxide
In the laboratory, sulphur dioxide is prepared by heating a mixture of sodium sulphite and dilute hydrochloric acid. The reaction equation is:

Alternatively, sulphur dioxide can be prepared by heating a mixture of concentrated sulphuric acid and copper. In this case, there is no reaction until the mixture in the flask becomes hot. Then rapid effervescence occurs and the gas is usually collected as shown.

A dark brown mixture is left in the flask. It contains anhydrous copper (II) sulphate and certain impurities.
The Properties of Sulphur Dioxide
Describe the properties of sulphur dioxide
Physical properties
These are:
- The gas is colourless with an irritating (pungent), chocking smell.
- It is denser than air. Its density is 2½ times that of air.
- It is readily soluble in water and forms an acidic solution of sulphurous acid.

Acidic characteristics of sulphur dioxide
As indicated above, sulphur dioxide gas dissolves in water to form an acid solution of sulphurous acid, H2SO3. The solution turns blue litmus paper red. Sulphur dioxide is thus an acidic gas.
Chemical properties
The reaction characteristics described below explains the chemical properties of sulphur dioxide gas.
The reducing properties of sulphur dioxide
(i) Reduction of dyes in flower petals or colour in paper (bleaching)
Sulphur dioxide bleaches the colours in dyes such as flower pigments. When the flower pigments or dyes contain oxygen, they are coloured. Sulphur dioxide reduces the dye (removes oxygen from it) and the dye, therefore, turns colourless. This process can be summarized as follows:
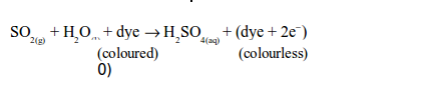
In this process, sulphur dioxide dissolves in water to form sulphurous acid:

Then the acid takes up oxygen from the dye of the flowers or paper and forms sulphuric acid. The removal of oxygen from the dye converts it to a colourless compound:

However, the oxygen from the air may oxidize the reduced colourless compound back to the original coloured compound.
(ii) Reduction of nitric acid
If sulphur dioxide gas is bubbled through concentrated nitric acid, brown fumes of nitrogen dioxide gas are formed:

The nitric acid is reduced to nitrogen dioxide and the sulphur dioxide is oxidized to sulphuric acid.
(iii) Reduction of acidified potassium permanganate solution
Sulphur dioxide decolourized purple potassium permanganate solution.
Sulphur dioxide is first converted to a sulphite (SO32-), after reacting with water, and then oxidized to a sulphate (SO42-) by the permanganate.

The ionic equation is:

The oxidation state of manganese changes from +7 to +2. This is a reduction reaction.
(iv) Reduction of acidified potassium dichromate solution
When sulphur dioxide is mixed with potassium dichromate (VI) solution the orange colour of the solution changes to green. The dichromate (VI) is reduced to chromate (III) which is green in colour when in aqueous state:
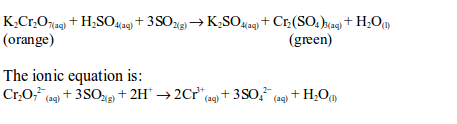
The oxidation state of chromium changes from +6 in potassium dichromate (VI) to +3 in chromic sulphate. On the other hand, sulphur dioxide is oxidized by the dichromate (VI) to sulphate (SO42-).
Other reduction reactions involving sulphur dioxide gas
Sulphur dioxide reduces chlorine, bromine and iodine to the hydrogen halides in the presence of water:
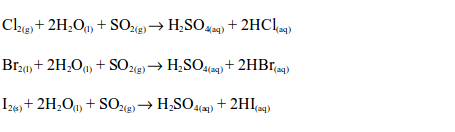
In all these cases, the solution changes from brown to colourless.
The oxidizing properties of sulphur dioxide
(i) Oxidation of hydrogen sulphide
Iron (II) sulphide reacts with dilute hydrochloric acid to produce hydrogen sulphide gas and iron (III) chloride:

Hydrogen sulphide gas has a smell similar to that of a rotten egg. The hydrogen sulphide gas is oxidized by sulphur dioxide in the gas jar to sulphur:

The sulphur produced is a yellow residue.The reaction takes place in the presence of moisture which acts as a catalyst. That is why water is added in the jar.
(ii) Oxidation of magnesium
When burning magnesium is lowered into a jar containing sulphur dioxide gas, a white solid, magnesium oxide, and yellow pieces of sulphur are formed:

Tests for sulphur dioxide gas
Includes
- The gas can be identified by its characteristic pungent and choking smell.
- It can also be detected by putting into it a filter paper that has been previously dipped into an acidified solution of potassium dichromate (VI). The colour of the filter paper changes from orange to green due to the reduction of dichromate (VI) to chromate (III).
- Sulphur dioxide also decolourized acidified potassium permanganate solution.
The Uses and Hazards of Sulphur Dioxide
Explain the uses and hazards of sulphur dioxide
Sulphur dioxide has got a number of uses in daily life. However, there are also some hazards which can be caused by the gas if its production is not controlled.
Uses of sulphur dioxide
The following are some uses of sulphur dioxide gas:
- The gas is used in the industrial manufacture of sulphuric acid in the Contact Process.
- It is used as a bleaching agent for wood pulp, silk, wool and straw.
- Its poisonous nature makes it a useful fumigant. So it is used in fumigation. The gas is poisonous to all organisms, particularly bacteria.
- It is used as a preservative and sterilizing agent in making soft drinks and jam, and in dried fruits. A very low concentration of the gas in food prevents fermentation as it stops the growth of bacteria and moulds. Its reaction with oxygen prevents oxidation of juices and other liquids to which it is added for preservation.
Hazards of sulphur dioxide gas
The hazards of sulphur dioxide gas are due to its effect in environmental pollution and the health problems accompanied with that pollution. Sulphur dioxide is a major air pollutant. The major sources of sulphur dioxide in the air are power plants that use fossil fuels such as coal, diesel and petrol; industrial boilers; and exhaust emissions from motor vehicles. The gas is also produced during metal smelting and other industrial processes.
Half of sulphur dioxide output comes from burning coal in coal-fired power stations. All coal contains small amounts of sulphur. So when the coal is burnt to produce energy, the sulphur in the coal reacts with oxygen in the air to produce sulphur dioxide

Sulphur dioxide is a very irritating gas and is thought to be the cause of bronchitis and other lung diseases. Exposure to higher concentrations of the gas can cause impairment of the respiratory function and heart diseases.
Sulphur dioxide also causes acid rain. This occurs when the gas comes in contact with moist air. The sulphur dioxide dissolves in water vapour from the clouds and combines with oxygen from the atmosphere to form an acid – sulphuric acid:

Acid rain damages the leaves and barks of plants making them more vulnerable to diseases, weather and insects. When acid rain reaches the lake, river or other water bodies it makes the whole water body acidic. Even a low concentration of acid in the water can kill fish and other marine organisms.
Sulphuric Acid
The Contact Process for the Manufacture of Sulphuric Acid
Describe the contact process for the manufacture of sulphuric acid
Sulphuric acid is an important laboratory and industrial reagent. It is produced in large scale through the Contact Process. The process involves four major stages. These are:
- production of sulphur dioxide;
- purification of sulphur dioxide;
- catalytic conversion of sulphur dioxide (SO2) to sulphur trioxide (SO3); and
- conversion of sulphur trioxide to sulphuric acid.
1. Production of sulphur dioxide
The sulphur dioxide used in the Contact Process can be obtained from different sources. These include:
(a) Burning sulphur in air:

This is the most convenient method of producing sulphur dioxide.
(b) Burning sulphide ores such as iron pyrite (FeS2) and zinc blend (ZnS):


Sulphur dioxide gas is produced as a by-product.
2. Purification of sulphur dioxide
The sulphur dioxide produced in the first stage is mixed with air, ready for passing it over the catalyst. Before contact with the catalyst is allowed, the gas mixture has to be purified to remove impurities. This is achieved by passing the mixture through an electrostatic precipitator to remove any dust. It is then washed with water to remove impurities such as traces of arsenic (III) oxide (As2O3). The gas mixture is then passed through concentrated sulphuric acid to remove all moisture. The three impurities (As2O3, dust and moisture), if not removed, will poison the catalyst thereby rendering it useless.
3. Catalytic conversion of sulphur dioxide to sulphur trioxide
The purified and dried mixture of sulphur dioxide and air is passed through a heat exchanger to acquire the necessary heat for the conversion to sulphur trioxide. The mixture is then taken to the conversion chamber, which contains a catalyst. The catalyst used is finely divided vanadium (V) oxide (vanadium pentoxide, V2O5) which is heated to 450°C.
Originally, platinized asbestos was used as a catalyst. But, compared to vanadium (V) oxide, platinum is very expensive and easily poisoned by impurities. So it has been replaced by vanadium (V) oxide as the usual catalyst used in the Contact Process.
Sulphur dioxide remains in contact with the catalyst during the conversion process, hence the name Contact Process. The reaction that takes place during the conversion is:
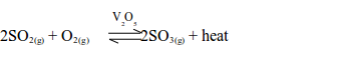
The conversion takes place at a temperature of 450°C and normal atmospheric pressure (1 atm).
The reaction is exothermic, which means that, as sulphur trioxide is formed, heat energy is released. If the temperature rises above 450°C the yield of sulphur trioxide decreases.
Once the reaction has started, no external heating is required. Thus, the heat exchanger maintains the temperature at 450°C. According to Le Chatelier’s principle, a lower temperature should be used to shift the equilibrium to the right, hence increasing the percentage yield. However, too low temperature will lower the formation rate to an economical level. Hence, to increase the rate, high temperature (450°C), medium pressure (1-2 atm) and a catalyst (V2O5) are used to ensure maximum yield. The catalyst only serves to increase the rate of reaction as it does not change the position of the dynamic equilibrium.
About 98% of the possible yield of sulphur trioxide is obtained.
4. Conversion of sulphur trioxide to sulphuric acid
The sulphur trioxide from the conversion chamber is passed through a heat exchanger to remove excess heat. It is then taken to an absorption tower where it is dissolved in concentrated sulphuric acid to form oleumor fuming sulphuric acid:

Oleum is then carefully diluted with the correct amount of water to give ordinary concentrated sulphuric acid:

Sulphur dioxide cannot be dissolved directly in water to form sulphuric acid. The reaction is so highly exothermic that the heat produced vapourizes the sulphuric acid formed. This makes it difficult to collect the gas because the acid vapour (mist) produced is very stable and cannot be condensed.
The flow diagram for the manufacture of sulphuric acid is show in the figure below
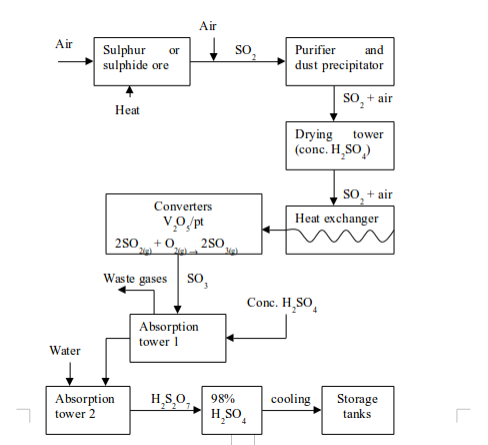
A flow diagram of the Contact Process
The Properties of Sulphuric Acid
Explain the properties of sulphuric acid
Chemical properties of dilute sulphuric acid
Dilute sulphuric acid reacts with metals, metal oxides, metal hydroxides and metal carbonates and hydrogencarbonates to produce salts.
Reaction with metals
Dilute sulphuric acid reacts with many metals above hydrogen in the activity series to form sulphates and hydrogen gas, e.g.
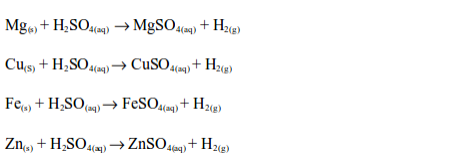
Reaction with metal oxides
Reactions of metal oxides with dilute sulphuric acid are neutralization reactions. Metal oxides react with dilute sulphuric acid to form a salt (sulphate) and water, e.g.
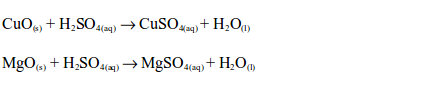
Reaction with metals hydroxides
The reaction between dilute sulphuric and a metal hydroxide is a neutralization reaction. Metal hydroxides react with dilute sulphuric acid to form a sulphate and water, e.g

Reaction with metal carbonates and hydrogencarbonates
Dilute sulphuric acid reacts with metal carbonates and hydrogencarbonates to give metal sulphates, carbon dioxide and water, e.g.
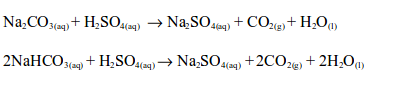
Chemical properties of concentrated sulphuric acid
Concentrated sulphuric acid as a dehydrating agent
As a dehydrating agent, it will remove the elements of water (hydrogen and oxygen) from a compound to form a new compound. It will dehydrate sugar, paper and wood. These are all made of carbon, hydrogen and oxygen. The acid removes the hydrogen and oxygen as water, leaving carbon behind.
When concentrated sulphuric acid is added to sugar, a vigorous reaction occurs, causing the reaction mixture to rise and fill the beaker.
The colour of the sugar changes to brown and finally black. Concentrated sulphuric acid dehydrates sugar (glucose) by taking away the elements of water (hydrogen and oxygen) from the sugar, leaving carbon.

The acid will also dehydrate sucrose to form carbon.

The final product is a black mass of carbon. The reaction is highly exothermic. The heat produced evaporates the water formed from the reaction.
The acid also dehydrates some hydrated salts. When concentrated sulphuric acid is added to hydrated blue copper (II) sulphate crystals, the colour changes from blue to white. The acid dehydrates the hydrated copper (II) sulphate crystals to anhydrous copper (II) sulphate powder:

Concentrated sulphuric acid also dehydrates methanoic acid and ethanol to give carbon monoxide and ethene gases respectively:
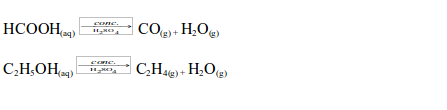
Concentrated sulphuric acid as a drying agent
As a drying agent, concentrated sulphuric acid absorbs traces of water from substances. Because of its ability to absorb water, it is used for drying most gases prepared in the laboratory that it would not react with. It cannot be used for drying ammonia, carbon dioxide, hydrogen sulphide or any gas with which it reacts.
Sulphuric acid as an oxidizing agent
Hot concentrated sulphuric acid is a strong oxidizing agent. It oxidizes both metals and non-metals while it is reduced to sulphur dioxide.
Concentrated sulphuric acid oxidizes charcoal (carbon) to carbon dioxide, sulphur to sulphur dioxide and copper to copper (II) sulphate.
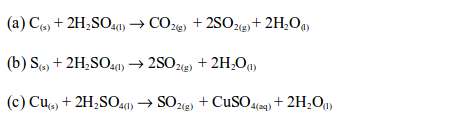
With sulphur and copper, the orange colour of the dichromate (VI) paper changes to green. This confirms the presence of sulphur dioxide gas.
With carbon, a white precipitate is formed on the glass rod when the rod dipped in lime water (calcium hydroxide) is placed in the mouth of the test tube. This confirms the presence of carbon dioxide gas which reacts with the calcium hydroxide on the glass rod to produce a white precipitate of calcium carbonate.

The Uses of Sulphuric Acid
Explain the uses of sulphuric acid
Sulphuric acid is one of the most important industrial chemicals. It has widely varied uses and plays some part in the production of nearly all manufactured goods. The following are some of the uses of sulphuric acid:
- Manufacture of fertilizers The major use of sulphuric acid is the production of fertilizers such as ammonium sulphate and superphosphates (phosphate fertilizers).
- Manufacture of chemicals It is widely used in the manufacture of chemicals e.g. in making hydrochloric acid, nitric acid, phosphoric acid, sulphate salts, synthetic detergents, soap, paints and pigments, explosives, plastics and drugs.
- Refining of crude oil A large quantity of sulphuric acid is used in refining crude oil.
- Extraction and manufacturing of metals Sulphuric acid is used in the iron and steel-making industry to remove rust and scale from the surface of the rolled iron sheets. It is also used in processing metals e.g. in pickling (cleaning) iron and steel before plating them with tin or zinc to produce galvanized iron.
- Manufacture of alum Sulphuric acid is used in the manufacture of aluminium sulphate, which is used in water treatment plants to filter impurities and to improve the taste of water. Aluminium sulphate is made by reacting bauxite with sulphuric acid.
- Manufacture of natural and man-made fibres Sulphuric acid is used for making natural and synthetic (artificial) fibres. For example, the textile called rayon is made from cellulose fibres derived from wood. These fibres are dissolved in a solution of tetraamminecopper (II) sulphate to produce a thick liquid which is then injected into sulphuric acid to form rayon fibres.
- Other uses:Sulphuric acid is used as (i) an electrolyte in lead-acid batteries, which are used in cars, to allow the flow of electrons between the plates in the battery. The sulphuric acid used in this way is called battery acid; (ii) as a general dehydrating agent in its concentrated form in tanning leather; and (iii) in waste water treatment.
Nitrogen
Occurrence
Nitrogen makes about 78% of the air by volume. The element also occurs combined with other compounds in the form of sodium nitrate, Chile saltpetre, NaNO3 (as a mineral deposit in Chile), and in the soil as ammonium sulphate, sodium nitrate, potassium nitrate and calcium nitrate.
Combined nitrogen also occurs as a constituent of all living matter of plants and animals in the form of proteins, enzymes, alkaloids.
Preparation of a sample of Nitrogen in the Laboratory
Prepare a sample of nitrogen in the laboratory
Nitrogen can be prepared in the laboratory by either separating the gas from the air or by heating ammonium nitrite.
(i) Preparation of nitrogen by the action of heat on ammonium nitrite
The reaction between sodium nitrite and ammonium chloride gives sodium chloride and ammonium nitrite.

A solution of ammonium nitrite readily decomposes, on slight warming, to give nitrogen gas.
As the solution becomes warm, rapid effervescence occurs as more nitrogen is given off.

The nitrogen evolved may be collected over water because the gas is only slightly soluble in water, at ordinary temperature, and slightly denser than air.
Tests for nitrogen gas
Aim: To test for the presence of nitrogen.
Procedure
Using the four gas jars of nitrogen gas collected in the previous experiment (Experiment 1.16), carry out the following tests for nitrogen gas and write down your observations:
- Remove the cover from the first jar and smell the gas. Observe the colour of the gas and identify its smell.
- Remove the cover from the second jar and put in it a piece of damp universal indicator.
- Place a lighted splint into the third gas jar.
- To the fourth jar, add some calcium hydroxide solution (lime water) and shake.
Observations and inferences
- The gas is colourless and odourless. This distinguishes it from gases such as sulphur dioxide, ammonia, hydrogen chloride, etc.
- The colour of the indicator does not change. This shows that nitrogen is a neutral gas.
- The lighted splint is extinguished and the gas does not burn. It can not, therefore, be any gas which supports combustion, e.g. oxygen, dinitrogen oxide, or any combustible gas, e.g. hydrogen sulphide, carbon monoxide, hydrogen, etc.
- After the above tests, the only gas with which nitrogen may be confused is carbon dioxide. To distinguish it from carbon dioxide, the gas is dissolved in calcium hydroxide solution. Nitrogen leaves the calcium hydroxide unchanged while carbon dioxide turns the solution milky (due to formation of a precipitate of CaCO3).
Properties of nitrogen
Physical properties
Nitrogen is colourless and odourless. It is slightly less dense than air and sparingly insoluble in water. The gas is neutral to litmus.
Chemical properties
Under ordinary conditions, nitrogen gas is inert. However, the gas only takes part in reactions at very high temperature as follows:
1. It reacts with red hot metals, like magnesium and calcium to form nitrites
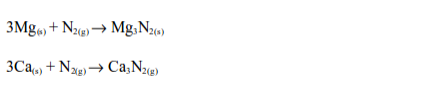
2. Nitrogen does not burn nor does it support combustion. When heated, the gas combines with oxygen to form nitrogen monoxide gas:

3. At ordinary pressure and moderately high temperatures, nitrogen reacts with hydrogen in the Haber Process to produce ammonia:

The Uses of Nitrogen
Explain the uses of nitrogen
Here are some uses of Nitrogen:
- Manufacture of fertilizers: Nitrogen is used to manufacture nitrogenous fertilizers. These include diammonium phosphate (DAP), calcium ammonium nitrate (CAN), ammonium superphosphate (ASP), ammonium nitrate (AN), ammonium phosphate sulphate (APS), ammonium sulphate nitrate (ASN), ammonium sulphate (AS) and urea.
- Refrigeration: Because of its low boiling point (-196oC), liquid nitrogen is used as a refrigerant for storing organs in research laboratories, bull semen for artificial insemination, eggs and other cells for medical research and reproductive technology, etc. It is also used for preservation of food products and for their transportation.
- Processing reactive substances:Some reactions require an inert atmosphere in order to proceed as desired. Because of its low reactivity, nitrogen is used to provide an inert atmosphere for storing and processing reactive substances.
- Manufacture of nylon: Nitrogen is used in the manufacture of synthetic fibres such as polyamides. Polyamides are commonly known as nylons. Nylons are chemically inert and are stronger than natural fibres. They are used in making fishing nets, clothes, ropes, and many other items.
- Manufacture of ammonia: Nitrogen is used in the manufacture of ammonia through the Haber Process.In the Haber Process, ammonia is manufactured by direct combination of nitrogen and hydrogen.Nitrogen and hydrogen are mixed in the ratio of 1:3. The gases are then reacted together at a temperature of about 450°C and a pressure of 250 atmospheres in the presence of finely divided iron as a catalyst. N2(g) + 3H2(g) ⇔2NH3(g) + heat The gases are cooled while still under pressure and ammonia is removed as a liquid.
- Manufacture of nitric acid: The ammonia gas manufactured using nitrogen in the Haber Process is used in the manufacture of nitric acid by catalytic oxidation.
- Plant nutrition: When atmospheric nitrogen is fixed into the soil by bacterial actions, it becomes a nutrient to plants. Nitrogen fixation refers to the conversion of atmospheric nitrogen, by certain species of bacteria, into nitrites.
Ammonia
Preparation of a Dry Sample of Ammonia Gas in the Laboratory
Prepare a dry sample of ammonia gas in the laboratory
Ammonia is a binary compound of nitrogen and hydrogen. Ammonia gas is colourless and has a strong pungent and choking smell. It does not occur free in air, but exists in nitrogenous organic materials such as hoofs and horns of animals. The gas can be released by heating or burning these materials.
Laboratory preparation of ammonia
Ammonia is prepared in the laboratory by heating any ammonium salt with an alkali. In most cases, ammonium chloride and calcium hydroxide (the cheaper alkali) are used. Both are solid so they must be thoroughly ground first to give a very fine mixture so that the reaction can occur efficiently.
Ca(OH)2(s) + 2NH4Cl(s)→CaCl2(s) + 2H2O(l) + 2NH3(g)
Calcium hydroxide reacts with ammonium chloride to produce ammonia gas, calcium chloride and water. The flask is tilted to prevent any condensed water formed from running back into the hot flask, which might break it.
Ammonia gas is dried by passing it over quicklime because it reacts with all the usual drying agents. Concentrated sulphuric acid is acidic and would absorb the gas forming a salt e.g. 2NH3(g) + H2SO4(l) → (NH4)2SO4(s)
It reacts with calcium chloride, forming solid complex compounds, e.g. 8NH3(g) + CaCl2(s)→ CaCl2.8NH3(s)
Ammonia is less dense than air and very soluble in water, so it is collected as shown by upward delivery (or downward displacement of air).
Ammonia is an alkaline gas and it turns wet, red litmus paper blue.
The Properties of Ammonia
Describe the properties of ammonia
Physical Properties of Ammonia
- It is a colourless poisonous gas with a strong chocking smell.
- It is less dense than air.
- It is easily liquefied by cooling to -33°C or by compressing it.
- It turns wet, red litmus paper blue as it is the only common alkaline gas
- It is very soluble in water forming alkaline solution. Ammonia has the highest solubility of all known gases. About 800 volumes of the gas dissolve in 1 volume of water at 15°C. The fountain experiment below demonstrates this solubility.
Chemical properties of ammonia
Reaction with air and oxygen
Ammonia will not normally burn in air but can be made to do so in two ways:
- Burning ammonia in an oxygen-rich atmosphere; and
- Use of a catalyst.
Burning ammonia in oxygen-rich air
When ammonia is burned in air enriched with oxygen, the products formed are nitrogen and water. 4NH3(g) + 3O2(g) → 2N2(g)+ 6H2O(l)
Using a catalyst
The source of ammonia gas in this experiment is concentrated ammonia solution which gives off fumes of ammonia gas. If some concentrated ammonia solution is left in a stoppered flask for a few minutes, the flask will quickly become full of ammonia gas by diffusion.
The catalyst used is the metal platinum. The platinum coil is heated in a bunsen flame until it is red hot. It is then lowered into a flask containing ammonia. The coil continues to glow even though it is not being heated. This indicates that a chemical reaction is taking place. Near the top of the flask brown fumes can be seen.
The products of this reaction are nitrogen monoxide (nitrogen (II) oxide), which is a colourless gas, and water.
4NH3(g) + 5O2(g) → 4NO(g) + 6H2O(g)
However, as the nitrogen monoxide gas moves towards the neck of the flask and comes into contact with the air, it reacts with the oxygen in the air to form nitrogen dioxide gas which is brown in colour. Hence, the brown fumes are seen at the neck of the flask.
2NO(g) + O2(g) → 2NO2(g)
Reaction with copper
When ammonia gas is passed over heated copper (II) oxide, the gas is oxidized to nitrogen and water by the hot copper (II) oxide while the oxide is reduced to copper.
3CuO(s) + 2NH3(g) → 3Cu(s) + 3H2O(l) + N2(g)
Copper (II) oxide is black. During the reaction it is oxidized to copper which is reddish brown in colour.
Reaction with hydrogen chloride gas
When ammonia and hydrogen chloride are mixed, dense white fumes of ammonium chloride are formed.
NH3(g) + HCl(g) → NH4Cl(s)
This test is simply performed by dipping a glass rod into concentrated hydrochloric acid then holding the glass rod at the mouth of a gas jar containing ammonia gas.
The Uses of Ammonia
Explain the Uses of Ammonia
Manufacture of fertilizer
Since ammonia solution is alkaline, it reacts with acids to form ammonium salts which are used as fertilizers.
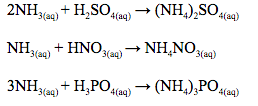
Other nitrogenous fertilizers manufactured using ammonia include ammonium sulphate nitrate (ASN), diammonium phosphate (DAP) and calcium ammonium nitrate (CAN)
Ammonia itself can be used directly as a fertilizer but has three disadvantages:
- Ammonia comes as a gas or a concentrated solution which is less easy to store; it is easier to store the solid ammonium salts.
- Ammonia is alkaline and can affect the natural pH of the soil.
- Ammonia easily evaporates if directly applied to the soil.
Manufacture of nitric acid
A lot of the ammonia from the Haber Process is used to make nitric acid. The raw materials for manufacture of nitric acid are ammonia, air and water.
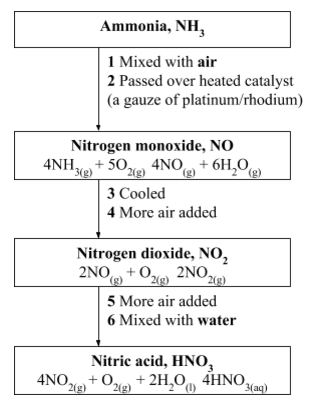
The overall result is that ammonia is oxidized to nitric acid.
Cleaning
Ammonia solution is very useful in cleaning. This is because it softens water in homes and laundries and neutralizes acid stains caused by perspiration, hence making washing easier. It is also used as a grease solvent.
Refrigeration
Liquid ammonia is used in large-scale refrigerating plants such as in ships and warehouses. It used to be used in domestic refrigerators, but has now been replaced by non-toxic, non-corrosive chlorofluorohydrocarbons (CFHs).
Manufacture of synthetic fibres
In the textile industry, ammonia is used in the manufacture of synthetic fibres such as nylon and rayon.
Carbon
The Forms in which Carbon Exists
Name the forms in which carbon exists
Although carbon forms less than one percent of the earth’s crust by weight, it is the most interesting of all elements. This is because of the following reasons:
- All living things are made of carbon and its compounds.
- Over ¾ of the world’s power is obtained from carbon and its compounds.
- Over ¾ of all substances in the world are made of carbon.
Carbon is not commonly found in a free state. The free element is mainly found as graphite and diamond. Carbon occurs in a number of other forms, e.g. wood charcoal, animal charcoal, coke, soot (lampblack).
Carbon compounds are found in many naturally occurring substances such as coal, petroleum, wood, coal gas, natural gas, carbonates, shells, organic matter of all kinds, all living things, and occurs in the air to a small, but very important extent (0.03 – 0.04% by volume) as carbon dioxide. The carbohydrates, proteins and lipids in all living things contain carbon.
Pure carbon is found in the form of diamond in Tanzania (Mwadui), Sierra Leone, India, South Africa, Russia and South America; and impure carbon, as graphite, is found in Sri Lanka.
Allotropic Forms of Carbon
Describe allotropic forms of carbon
An element that exists in more than one form is said to exhibit allotropy. Allotropy is the existence of an element in more than one form (without change of state). The various forms are known as allotropes.
Allotropes of a given element differ in their physical properties and may differ in some chemical properties as well. The allotropic forms of carbon are:
- graphite;
- diamond; and
- amorphous carbon.
These allotropes have got different molecular structures. The structural differences are mainly due to the way their atoms are packed.
Graphite
Graphite has a layer structure. Figure 1.18 illustrates one layer of the structure of graphite. Each layer consists of carbon atoms covalently bonded together into hexagonal rings. These rings form flat parallel layers, one over the other. The force that hold the carbon atoms together are very strong. Adjacent layers are held together by weak van der Waals’ forces as shown in figure 1.19. The layers readily slide over one another, accounting for the soft and greasy texture of graphite.
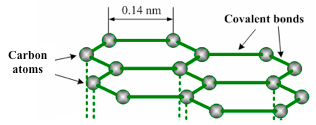
Carbon atoms in one graphite layer
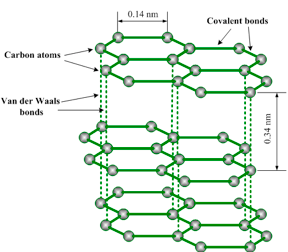
Graphite structure
Carbon atom has got four electrons in its outer shell. Each carbon atom forms three covalent bonds to other carbon atoms. Thus, three of its four outermost electrons are paired up to form covalent bonds. The fourth electron is not attached to any particular atom (delocalized) and is free to move anywhere along the layers. Graphite conducts electricity in the plane of the layers but not at right angles to them.
Because conduction of electricity involves movement of unshared electrons from one atom to another atom, graphite is a good conductor of electricity since the hexagonal layers permit this movement. It is also a good conductor of heat for a similar reason.
Physical properties of graphite
- It is a black, soft and slippery substance. It feels soapy and greasy. It has a metallic lustre and is opaque to light.
- It has low relative density (2.3) as compared to diamond (3.5)
- Graphite is a good conductor of heat and electricity due to the delocalized electrons.
- It has a very high melting point (3730°C) and boiling point (4830°C). The melting and boiling points are high because of strong covalent bonds between the carbon atoms which require more energy (heat) to break in order to melt graphite.
Uses of graphite
- It is used as an electrode in electrolysis and as a positive terminal in dry cells. The use of graphite as electrode in electrolysis has an advantage because it does not react readily with most substances (it is an inert electrode).
- It is used as a lubricant, particularly when high temperatures are involved, where the usual lubricating oils easily decompose due to extreme heat. It is a lubricant for dynamos, electric motors and fast-moving parts of machinery.
- The major use of graphite is in making lead pencils of different hardness, by mixing it with different proportions of clay. The weakly held layers of carbon atoms in graphite easily slide over each other and are left behind on paper as black marks.
- Being resistant to chemicals and having a high melting point and also because it is a good conductor of heat, graphite is used to make crucibles.
- Graphite has the ability to absorb fast-moving neutrons, thus, it is used in nuclear reactors to control the speed of the nuclear fission reaction.
Diamond
The basic unit of the diamond structure is shown in figure 1.20. Each carbon atom is covalently bonded to four other carbon atoms. This basic unit is repeated in three dimensions as shown in figure 1.21 to form a giant tetrahedral structure of millions of carbon atoms, all forming four covalent bonds to each other. The melting point of diamond is high. This is because of the strong covalent bonds between carbon atoms, which require a large amount of heat energy to break up.
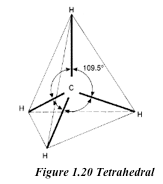
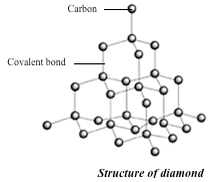
Physical properties of diamond
- It is the hardest natural substance known. This due to the strong covalent bonds between the carbon atoms in diamond. Again the compact tetrahedral arrangement of carbon atoms contributes to its hardness.
- It has the highest melting point (3550°C) and boiling point (4289°C).
- It has a high relative density (3.5) compared to graphite (2.3)
- It is a poor conductor of heat and electricity. This is because there are no free electrons to conduct electricity. All electrons are firmly held in covalent bonds.
- It is colourless, transparent and has a dazzling (amazing) brilliant lustre and radiance.
- It has a high refractive index of 2.5. The high refractive index results in high dispersion of light, making it suitable for use in jewellery.
Uses of diamond
- It is used in making jewellery.
- Due to its extreme hardness, it is used to make glass cutters, drilling devices, rock borers, and as an abrasive for smoothing very hard materials.
Comparison of the properties of diamond and graphite
Diamond | Graphite |
1. Colourless, transparent andglittering | Black, opaque with metallic lustre |
2. Hardest natural substanceknown, used to cut glass andin drills | Soft to touch, greasy or soapy, can be used as a lubricant and in making lead pencils |
3. High relative density (3.5) | Low relative density (2.3) |
4. Non-conductor | Good conductor of heat and electricity |
5. Burns in air least readily (atabout 900°C) | Burns in air readily (at 700°C) |
6. Have strong C-C covalentbonds arranged octahedrally toform a giant molecular crystal | Have strong C-C bonds within the hexagonal rings in the sheets but weak Van der Waal’s forces in between layers. |
7. Its cleavage is difficult and itoccurs along the boundaries ofthe octahedral crystal unit | Cleavage easy, and occurs along the sheets or the layers. |
8. Prepared from graphite at very high pressure and temperature | Prepared from coke and silica mixture at high temperature |
9. Not attacked by potassiumchlorate and nitric acid together | Attacked by these reagents |
A proof that diamond and graphite are both allotropic forms of carbon can be shown experimentally by burning equal masses of each allotrope in a stream of oxygen. It is found that equal masses of each allotrope produce equal masses of carbon dioxide. C(s)+ O2(g) → CO2(g)
The weight of carbon dioxide produced can be determined by allowing it to be absorbed in a weighed amount of potassium hydroxide solution. 2KOH(aq) + CO2(g) → K2CO3(aq) + H2O(l). Then, the weight of carbon dioxide is obtained by calculations based on the equation above.
Amorphous carbon
Amorphous carbon is carbon that does not have any clear shape, form or crystalline structure. Amorphous carbon is made of tiny bits of graphite with varying amounts of other elements considered as impurities. It is formed when a materials containing carbon is burned in a limited supply of oxygen, resulting in incomplete combustion.
Amorphous carbon exists in many forms. The following are the major forms in which it occurs:
- Charcoal
- Lampblack (soot) or carbon black
- Coke
Charcoal
Charcoal is made by heating organic material (animal or plant parts) to a high temperature in the absence of air or in the presence of limited amounts of oxygen or other reagents, catalysts, or solvents. This process is called destructive distillation. There are three categories of charcoal, namely, wood charcoal, animal charcoal and sugar charcoal.
Wood charcoal
Wood charcoal is made by heating wood or other vegetable matter (for example coconut shells) in the almost complete absence of air. Wood charcoal is light, porous and is a remarkably good absorbent for liquid or gases (1 cm3 of wood charcoal will absorb 100 cm3 of ammonia gas at 0°C).
Uses of wood charcoal
- Because of its ability to absorb large amounts of gas or liquid, it is used in gas masks to absorb poisonous gases in air in industrial process to recover volatile materials from waste gases.
- Wood charcoal can be used in metal refining instead of coke.
- Wood charcoal is a good source of energy. Thus, it is used as fuel for cooking and heating in homes.
Animal charcoal
Animal charcoal is made by heating animal bones in the absence of air. Its main component is calcium phosphate, Ca3(PO4)2, and carbon constitutes about 10% of the components.
Uses of animal charcoal: It is used in sugar industries to remove the colouring matter from brown sugar to make it white
Sugar charcoal
Sugar charcoal is a very pure form of carbon, and is made by removing the elements of water (oxygen and hydrogen) from sugar. This is achieved by using strong dehydrating agents such as concentrated sulphuric acid or concentrated nitric acid, which removes water from sugar.
Uses of sugar charcoal: Sugar charcoal is chiefly used in the manufacture of artificial diamonds.
Lampblack/soot/carbon black
This is produced by burning petroleum or any hydrocarbon in a limited supply of air (or in chlorine). It can also be produced from the burning of organic material at home. It is commonly found in the kitchen chimneys, lamps and on bases of cooking pans and pots.
Uses of lampblack/soot/carbon
- It is used in making printers’ ink, shoe polish and carbon papers.
- It is an important industrial material in the manufacture of tyres. It is used as a filler material in tyres.
Coke
Coke is made by destructive distillation of coal. During the process, coal is obtained as the major product. The other products formed when coal is destructively distilled are coal gas, coal tar, coal oil, gas carbon and ammonia liquor.
Uses of coke
- Coke is used as a fuel and as a reducing agent in the extraction of iron, lead and zinc. It is also used as a fuel in boilers.
- It is used in the manufacture of producer gas and water gas.
Chemical properties of carbon
- Carbon burns in excess oxygen to form carbon dioxide C(s) + O2(g) → CO2(g).In insufficient oxygen, carbon monoxide is formed.2C(s) + O2(g) → 2CO(g)
- Carbon has got reducing properties and thus acts as a reducing agent. Carbon reduces oxides of metals below it in the electrochemical and activity series to their respective metals. This occurs on strong heating, and this reaction is used industrially for extraction of metals from their ores:PbO(s) + C(s) → Pb(s) + CO(g);Fe2O3(s) + 3C(s) → 2Fe(s) + 3CO(g);ZnO(s) + C(s) → Zn(s) + CO(g).
- Sulphur vapours react with red hot carbon to give carbon disulphide. C(s) + 2S(g) → CS2(l)
- Carbon dioxide is reduced by red hot carbon to carbon monoxide C(s) + CO2(g) → 2CO(g);This reaction is used in the industrial manufacture of producer gas.
Carbon Dioxide
Preparation of a Dry Sample of Carbon Dioxide Gas in the Laboratory
Prepare a dry sample of carbon dioxide gas in the laboratory
Carbon dioxide is one of the oxides of carbon. The gas is present in the air at a level of approximately 0.03% by volume. It is also found dissolved in water. The gas is one of the by-products of all decaying organic matter. Without carbon dioxide there is no life on earth. It is used by all plants in the process of photosynthesis and both plants and animals evolve carbon dioxide in respiration.
Laboratory preparation of carbon dioxide
Carbon dioxide is prepared in the laboratory by the action of dilute hydrochloride acid on marble (calcium carbonate).
When dilute hydrochloric acid is poured on marble chips, effervescence occurs. Dilute hydrochloric acid reacts with the marble chips to give calcium chloride, water and carbon dioxide.
CaCO3(s) + 2HCl(aq) → CaCl2(g) + H2O(l) + CO2(g)
The Properties of Carbon Dioxide
Analyse the properties of carbon dioxide
Physical properties
- Carbon dioxide is a colourless and odourless gas.
- It is denser than air.
- When the gas is cooled to –78°C, it turns straight into the solid (it sublimes). Sublimation is the change of a gas straight into a solid or change of a solid straight into a gas. Solid carbon dioxide is called dry ice. It sublimes when it is heated or exposed to air.
- It has a melting point of –199°C and boiling point of –91.5°C.
- Carbon dioxide does not support combustion. This is why it is used in fire extinguishers.
Chemical properties
Reaction of carbon dioxide with lime water (Test for carbon dioxide)
When a little carbon dioxide gas is bubbled into lime water (calcium hydroxide solution), the solution turns milky. This is due to the formation of a white precipitate of insoluble calcium carbonate.
Ca(OH)2(aq) + CO2(g) → CaCO3(s) + H2O(l)
This is a confirmatory test for the presence of carbon dioxide. The test serves to distinguish carbon dioxide from any other gas.
When excess carbon dioxide is bubbled into the lime water, the white perceptible dissolves to form a clear solution of soluble calcium hydrogen carbonate: CaCO3(s) + H2O(l) + CO2(g) → Ca(HCO3)2(aq)
Barium hydroxide can also be used to test for carbon dioxide as it forms a precipitate of barium carbonate: Ba(OH)2(aq) + CO2(g) → BaCO3(s) + H2O(l)
Reaction of carbon dioxide with magnesium
When a burning magnesium ribbon is lowered into a gas jar containing carbon dioxide gas, it continues to burn for a short time with a spluttering flame. A white ash of magnesium oxide and black specks of carbon are formed. The black specks of carbon can be seen on the sides of the gas jar.
2Mg(s) + CO2(g) → 2MgO(s) + C(s)
This clearly shows that carbon dioxide contains carbon and oxygen.
Reaction of carbon dioxide with water
Carbon dioxide reacts with water to form a weak carbonic acid. When carbon dioxide is bubbled into water, it dissolves to form a weakly acidic solution of carbonic acid:
H2O(l) + CO2(g) ⇔ H2CO3(aq)
The solution turns a blue litmus paper pink. This indicates that the solution is slightly acidic and hence too weak to turn the blue litmus paper to red (as strong acids do). The solution has no effect on red litmus paper.
The Uses of Carbon Dioxide
Explain the uses of carbon dioxide
Uses of Carbon Dioxide include:
- Fire extinguisher: Carbon dioxide is inert (i.e. it does not burn). It is dense than air and does not support combustion. Hence, it is a very useful fire-fighting chemical. When applied to fire, it forms a blanket over the burning material. Thus, it prevents air (oxygen) from reaching the burning material and therefore, extinguishing the flames.
- Manufacture of aerated (fizzy) drinks: Soda water and mineral water contain carbon dioxide dissolved under pressure. Because the gas is only slightly soluble, it is bubbled into these drinks under pressure to make more of it dissolve. When the bottles are opened, the gas escapes and it causes the “fizzy”.Dissolved carbon dioxide is responsible for the pleasant taste of soft drinks such as lemonade, Coca cola, Pepsi cola and other aerated drinks and mineral waters. Other beverages like beers are also bottled together with carbon dioxide.
- Refrigeration: Carbon dioxide is used for refrigeration purposes (i.e. in the deep-freezing of foods). The gas liquefies at ordinary pressure to form dry ice which sublimes at -78°C. Dry ice is a good refrigerant because it leaves no liquid after sublimation as is the case with ordinary ice.
- Manufacture of sodium carbonate by the Solvary Process:Carbon dioxide is used in the manufacture of anhydrous sodium carbonate in the Solvary Process. The sodium carbonate produced is used in the manufacture of glass.
- Manufacture of baking soda: Carbon dioxide is used in making baking soda (sodium bicarbonate). Baking soda is prepared by passing carbon dioxide into cold concentrated sodium hydroxide solution: CO2(g) + 2NaOH(aq)→ Na2CO3(aq) + H2O(l).Further addition of carbon dioxide produces sodium bicarbonate which, at sufficiently high concentration, will precipitate out of the solution as a solid: Na2CO3(aq) + CO2(g) + H2O(l) → 2NaHCO3(s) Yeast and sodium bicarbonate (hydrogencarbonate) are important in the baking industry. Thus in baking of bread, yeast is added to flour, sugar and water (forming the dough). In the making of cakes, baking powder (a mixture of bicarbonate and an acid) is used instead of yeast.
- Rain making: When pieces of dry ice (solid carbon dioxide) are dropped into clouds, the temperature of the clouds is lowered to such an extent that rain precipitates out.
- Photosynthesis: Plants use carbon dioxide from the air to manufacture their own food through the process of photosynthesis
TOPIC 2: ORGANIC CHEMISTRY
Introduction to Organic Chemistry
Organic chemistry is the chemistry of carbon compounds. Due to the ability of carbon to form chains of atoms, and for other atoms or groups of atoms to be attached to these chains, there are a huge number of carbon compounds. All organic compounds contain carbon together with one or more other elements such as hydrogen, oxygen, nitrogen, sulphur and the halogens.
Normally every compound of carbon is an organic compound. Even after discovering that these compounds could be synthesized in the laboratory, the definition that they are organic (of organic nature, that is, they originate from living things) has been retained. However, for conventional and historic reasons, some of the simpler compounds such as carbon dioxide (CO2) carbonates, carbon monoxide (CO3) are usually studied with other non-carbon compounds in Inorganic Chemistry.
Difference between Organic from Inorganic Chemistry
Distinguish organic from inorganic chemistry
Organic chemistry is the chemistry of carbon compounds. All organic compounds contain carbon and other elements such as H, O, N, S and the halogens. Normally every compound of carbon is an organic compound. Examples of organic compounds/substances are plastics, milk, carbohydrates, lipids, proteins, sugar and hydrocarbons. Inorganic substances includes table salt, CO2, diamond, iron and water.
The Importance of Organic Chemistry in Life
Explain the importance of organic chemistry in life
Carbon is the most unusual atom. It has the ability to join up to itself and form very long chains of atoms. Without this ability, life on Earth would not exist because the molecules that make our bodies contain mostly long chains of carbon atoms.
All living things contain carbon compounds. Raw materials such as oil and coal, derived from living things, are also based on carbon. Our modern society is very much dependent on organic chemistry to make the fuels and materials that we use in every day of our lives. In particular, polymers, large molecules obtained from alkanes, have very widespread use. Without alkanes from crude oil our transport system would be impossible.
We also need various fractions obtained from crude oil (petrol, diesel, kerosene, oil, natural gas, etc.) to run motor vehicles and other machines to simplify our work and life.
In brief, the compounds obtained from crude oil have thousands of different uses, for example:
- some are used as fuel or converted into fuels;
- some are used for making detergents, dyes, drugs, paints, and cosmetics; and yet some are used for making polyethene, polyvinyl chloride (PVC) and other plastics.
The Origin of Organic Compounds
Explain the origin of organic compounds
Fossil fuels were formed in the Earth’s crust from material that was once living. Coal comes from fossils of plant material. Crude oil and natural gas are formed from bodies of marine microorganisms. The formation of these fuels took place over geological periods of time (many millions of years).
Crude oil is one of the world’s major natural resources. The oil is the result of a process that began up to 400 million years ago. Prehistoric marine organisms died and sunk to the sea bed and were covered by mud and sand. The change into crude oil and natural gas was brought about by high pressure, high temperature and bacteria acting over millions of years. The original organic material broke down into hydrocarbons. Compression of the mud above the hydrocarbon mixture transformed it into shale. Then geological movements and pressure changed this shale into harder rocks, squeezing out the oil and gas. The oil and gas moved upwards through the porous rocks, moving from high- pressure to low-pressure conditions. Sometimes they reached the surface, but often they became trapped by a layer of non porous rock. Reservoirs of oil and gas were created. These reservoirs are not lakes of oil or pockets of gas. Instead, the oil or gas is spreadthroughout the pores in coarse rocks such as sandstone or limestone the same as water is held in a sponge.
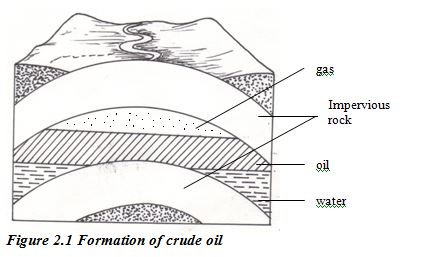
The Fractional Distillation of Crude Oil
Describe the fractional distillation of crude oil
Crude oil from an oilfield is separated from impurities such as sand and water and is pumped through pipelines to the refinery. At the refinery, fractional distillation is used to separate the crude oil into several fractions, each fraction containing several hydrocarbons which boil within a certain range of temperatures. These different boiling points are roughly related to the number of carbon atoms in the hydrocarbon (Table 2.1)
Separation of the hydrocarbons takes place in a fractionating column (fractionating tower). At the start of the refinery process, crude oil is preheated to a temperature of 350–400°C and pumped in at the base of the tower. As it boils, the vapour passes up the tower. It passes through a series of bubble caps and cools as it rises further up the column. The different fractions cool and condense at different temperatures, and therefore at different heights in the column. The fractions condensing at the different levels are collected on trays.
Thus, vapour is rising and liquid falling at each level in the tower. As a result very efficient fractionation occurs. Liquid is taken off at several different levels, the higher the level, the lower the boiling point of the fraction removed. Figure 2.2 shows the process of separation of crude oil into different fractions.
After fractional distillation, impurities are removed. The commonest impurity is sulphur, which is removed and used to manufacture sulphuric acid. If petrol (gasoline) containing sulphur is not purified before it is used in an internal combustion engine, the exhaust fumes will contain oxides of sulphur (SO2 and SO3). These are poisonous gases and will pollute the environment.
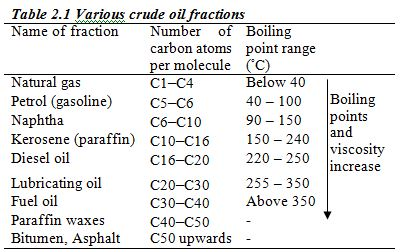
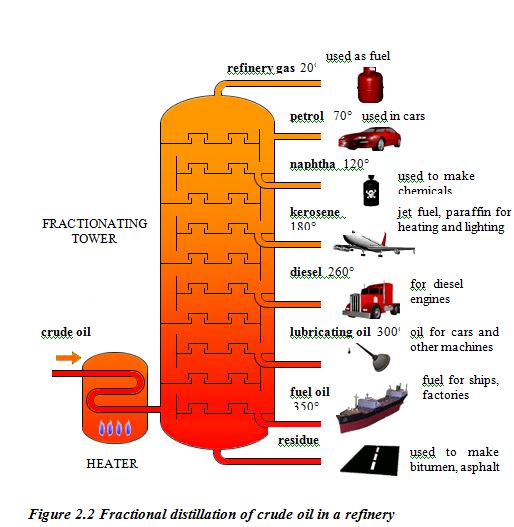
Uses of different petroleum fractions
1. Natural gas (refinery gas). The gas fractions consist of mainly methane, ethane, propane, and butane. The methane and ethane are usually burnt as fuel. The propane and butane are liquefied and are distributed in high pressure gas cylinders and tanks to be used for lighting and heating purposes.
2. Petrol (motor gasoline). It is mainly used as a fuel in internal combustion engines in motor vehicles. It is also used as a solvent for grease stains and paints.
3. Naphtha. It is used as a raw material for making many chemicals and plastics.
4. Kerosene (paraffin). It is used in homes as a fuel in paraffin lamps and stoves for heating, lighting and cooking food. However, in addition to its domestic use, it is used as a fuel for jet engines in aeroplanes. It is also used as a detergent.
5. Diesel oil. It is used as a fuel in diesel engines (e.g. in diesel train engines, tractors, lorries, diesel car engines).
6. Lubricating oil. It is used to make petroleum jelly (e.g. Vaseline). It is also used as oil for lubricating moving parts of cars and other machines.
7. Fuel oil. It is used as a fuel for power stations, ships and factories.
8. Paraffin waxes. They are used to make candles, polishes and waxed papers. They are also used in water proofing and as grease.
9. Asphalt, bitumen. They are used to make protective coatings for road surfaces and concrete roof tops, and also as binding agents for roofing sheets.
Hydrocarbons
Hydrocarbons are compounds containing hydrogen and carbon and no other element. That is, a hydrocarbon has the molecular formula CxHy, x and being whole numbers. For example, methane (CH4) ethane (C2H2) and benzene (C6H6) are hydrocarbons.
The three Families of Hydrocarbons
Classify the three families of hydrocarbons
In order to simplify the study of these compounds, chemists have grouped them into families. The members of each family have characteristic chemical properties and graded physical properties, such as boiling point, etc. The main family members of hydrocarbons that we shall study at this level are the alkanes, the alkenes and the alkynes.
Alkanes
The members of this group of hydrocarbons are distinguished by possessing the general molecular formula Cn H2n+2, where is 1, 2, 3, etc., for successive members of the group. The first member of the series (n = 1) is methane (CH4) and the second (n = 2) is ethane (C2H6). Both are gases at room temperature and pressure. This general formula can be used to work out the formula of any other alkane if we know the number of carbon atoms it consists of. The following table gives the molecular formula and name of the first few alkanes, plus an indication of some of their physical properties.
Table 2.2 Formulae and physical properties of some alkanes
name | Molecular formula | Melting point (oC) | Boiling point (oC) | Density (gcm–3) |
Methane | CH4 | –183 | –162 | gas |
Ethane | C2H6 | –172 | –89 | gas |
Propane | C3H8 | –188 | –42 | gas |
Butane | C4H10 | –135 | –1 | gas |
Pentane | C5H12 | –130 | 36 | 0.626 |
Hexane | C6H14 | –95 | 69 | 0.659 |
Heptane | C7H16 | –91 | 98 | 0.684 |
Octane | C8H18 | –57 | 126 | 0.703 |
Nonane | C9H20 | –54 | 151 | 0.718 |
Decane | C10H22 | –30 | 174 | 0.730 |
Structure of alkanes
In the alkanes, all carbon atoms show a covalency of four. Table 2.3 shows the structures of the first five members of the alkanes.
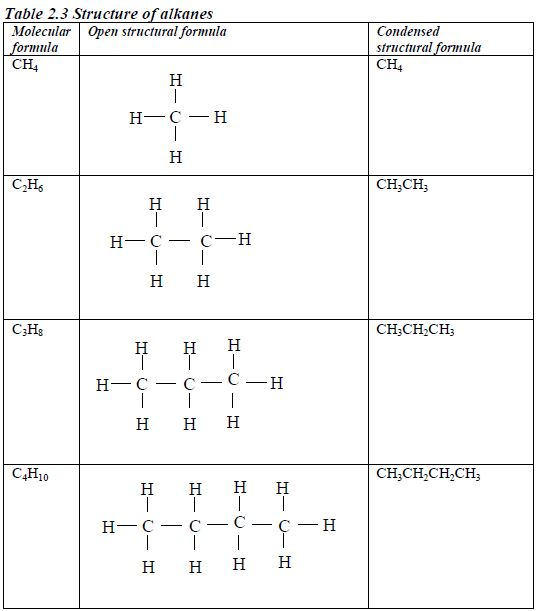
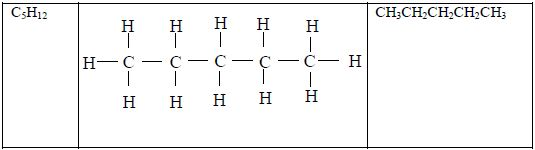
The Homologous Series of the Three Families of Hydrocarbons
Write the homologous series of the three families of hydrocarbons
A homologous series is a series of compounds with similar chemical properties which differ by –CH2. Such series has the following characteristics:
- All members obey the general molecular formula, e.g. for alkanes Cn H2n+2, alkenes Cn H2n and alkynes, Cn H2n-2.
- Each member differs, in molecular formula, from the next by CH2, e.g. alkanes are CH4, C2H6, C3H8, and so on.
- All members show similar chemical properties.
- The general properties of members change gradually in the same direction along the series, e.g. in alkanes, boiling points and freezing points rise with increase in the number of carbon atoms (CH4 – a gas; C5H12 – a liquid; C20H42 – a solid at ordinary temperatures and pressure).
- General methods of preparation are known which can be applied to any member of the series. Other homologous series are alcohols, CnH2n+1 and carboxylic acids, CnH2n+1COOH.
Nomenclature of alkanes
The term nomenclature means naming. Organic compounds are named using IUPAC system of nomenclature. IUPAC stands for International Union of Pure and Applied Chemistry.
Rules of Nomenclature
1. Name the longest unbranched carbon chain. For example, consider the following compound:
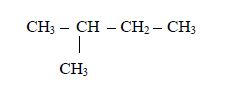
In this compound, the longest unbranched chain is
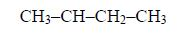
The longest chain is, therefore, butane.
2. Name the functional groups. Functional groups are branches of the main carbon chain. These are sometimes called alkyl groups.
Examples of alkyl groups are as follows:
- CH3 – methyl
- CH3CH2 – ethyl
- CH3CH2CH2 – propyl
- Cl – chloro
- Br – bromo
- I – iodo
- F – flouro
3. Give the position(s) of the functional group(s) using lower numbers when possible. Consider the following example:
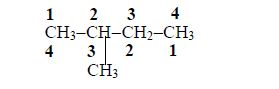
There are two possibilities of naming the above compound but only one is correct.
- 2 – methylbutane
- 3 – methylbutane
The first name is correct because it bears the lowest number (2). The second name is wrong because it bears a large number (3).
4. Names of the functional groups are named in alphabetical order in the final name.
Example
Consider the following compound:
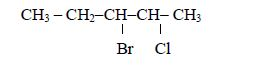
The name of the compound is: 3–bromo–2–choloropentane
Note: We start with bromo and not chloro because b comes before c in alphabet (a, b, c, d …)
5. If there are identical functional groups, use the prefixes di (2), tri (3), tetra (4), penta (5), etc. These prefixes do not account for alphabetical order.
Examples:
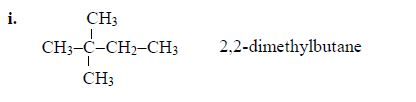
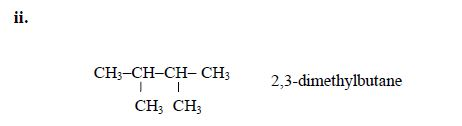
6. Commas separate numbers and hyphens separate numbers from words.
The Concept of Isomerism
Explain the concept of isomerism
Isomers are organic compounds with the same molecular formula but different structural formulae.
Isomerism is the occurrence of two or more compounds with the same molecular formula but different molecular structures (structural formulae).
Isomers of the same molecular formula have different physical and chemical properties because of structural differences.
Structural Formulae of all Isomers of Hydrocarbons up to Five Carbon Atoms
Write structural formulae of all isomers of hydrocarbons up to five carbon atoms
Example 1
1. Butane The two isomers of butane, with molecular formula C4H10, are as shown below. Isomers can be presented either in an open formula (structure) as follows.
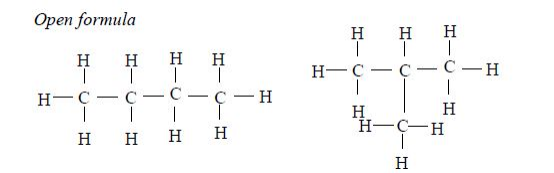

Example 2
Pentane
The three isomers of pentane showa open formula asfollows:
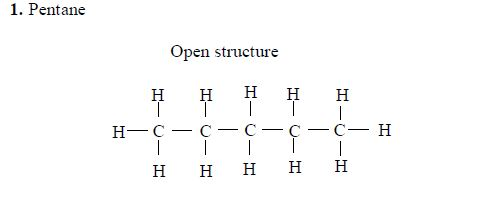
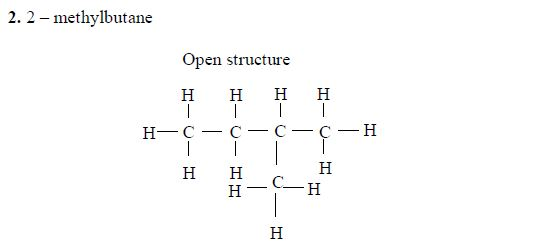
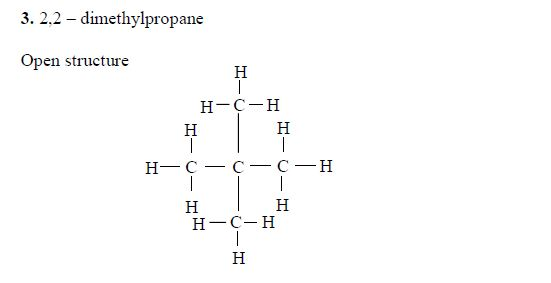
Isomers of Hydrocarbons up to Five Carbon Atoms
Name the isomers of hydrocarbons up to five carbon atoms
Example 3
alkanes showing isomerism
1. Butane The two isomers of butane, with molecular formula C4H10, are as shown below. Isomers can be presented either in an open or condensed formula (structure).
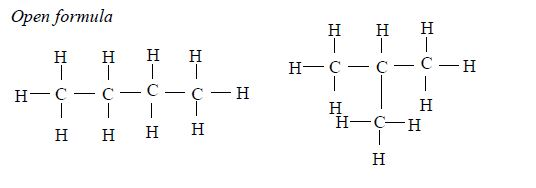
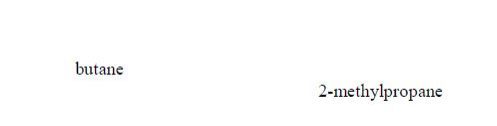
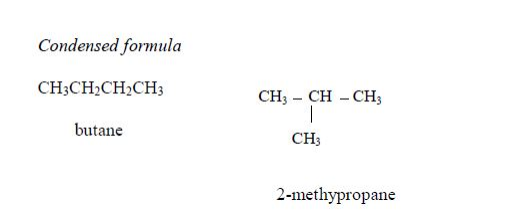
Note: Remember that CH4, C2H6 and C3H8 have no isomers because they cannot form alkyl groups, that is, they cannot be branched. Isomerism in alkanes starts from an alkane with four carbon atoms (butane) in its structure (C4H10).
2. Pentane
The three isomers of pentane are shown as follows:
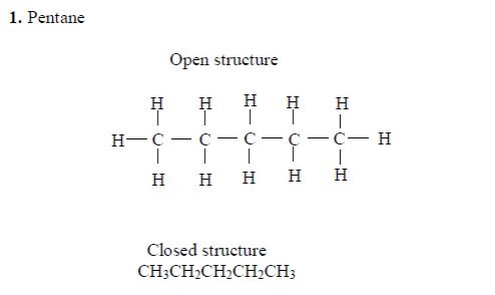
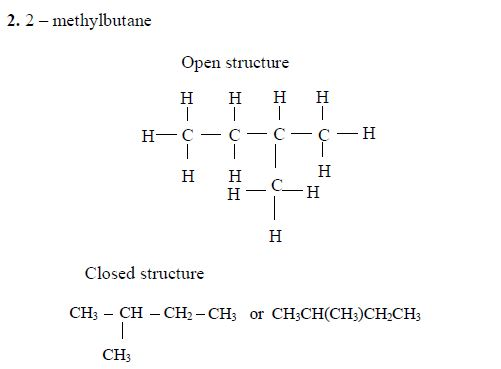
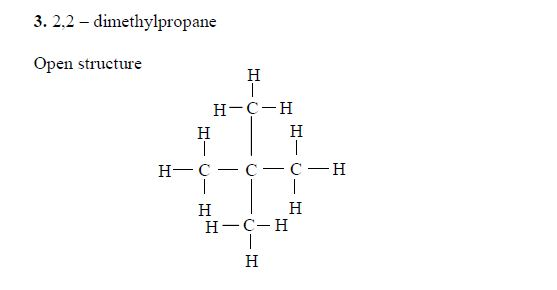
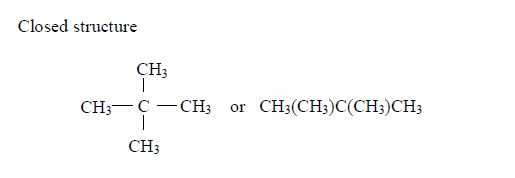
Alkenes
Alkanes are members of a homologous series of hydrocarbons with general molecular formula Cn H2n where n = 2, 3, 4, etc. The common structural feature is the presence of a carbon–carbon double bond:
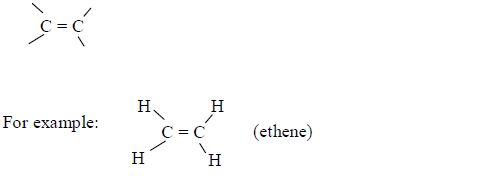
There is no member of this series for n = 1 since there needs to be at least two carbon atoms present to have a double bond. Table 2.3 shows the first five members of alkenes.
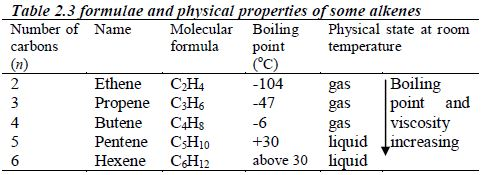
Structure of alkenes
Alkanes are characterized by possessing one or more carbon to carbon double bond(s) (C=C) in the carbon chain. Alkenes are unsaturated hydrocarbons because it is possible to break the double bond and add extra atoms to the parent molecule.
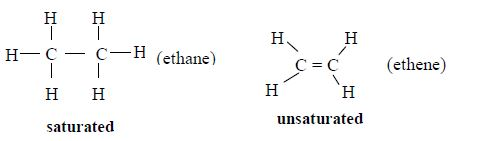
Table 2.4 shows the structures of the first five members of the homologous series of alkenes.
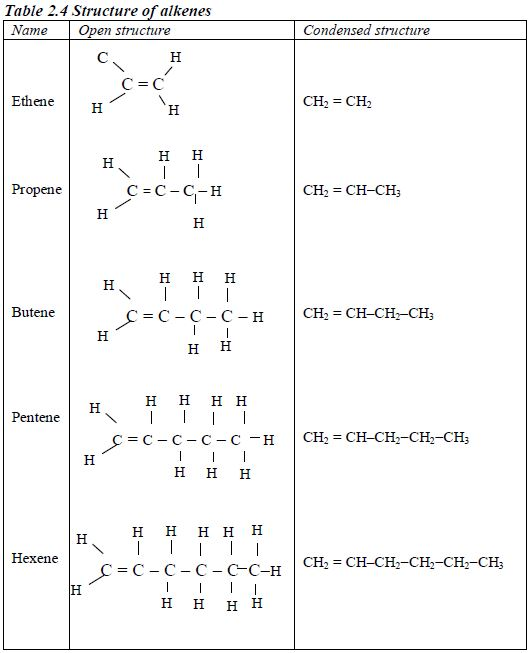
Nomenclature of alkenes
Rules for naming alkenes
- Names of alkenes have the ending –ene.
- The double bond is given the number of the carbon atom where it begins.
- In addition to the rules discussed earlier, the position of the double bond must be included in the name .Examples: CH3–CH=CH3 prop-1-ene , CH3–CH=CH–CH3 but-2-ene
- Double bonds are given the lowest number possible, usually lower than the functional groups.
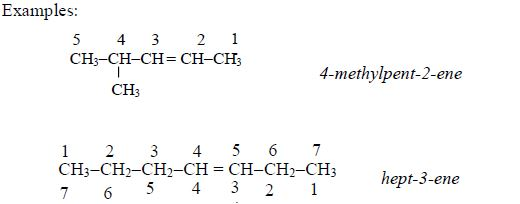
Counting from the left to right, the double bond (=) is on carbon number 4 and from the right to left, it is on carbon number 3. Because the double bonds should be given the lowest umber possible, the name of the compound is, therefore, hept-3-ene instead of hept-4-ene.
Isomerism
Alkenes, like alkanes show branching isomerism, for exampleCH3–CH=CH–CH3 (pent-1-ene) can be branched into (3-methylbut-1-ene) as follows:

They also show positional isomerism due to different positions of the double bond in the molecule, for example, pent-1-ene and pent-2-ene are both the isomers of pentene (C5H10):
CH2=CH–CH2–CH2–CH3 pent-1-ene
CH3–CH=CH–CH2–CH3 pent-2-ene
Other examples include:
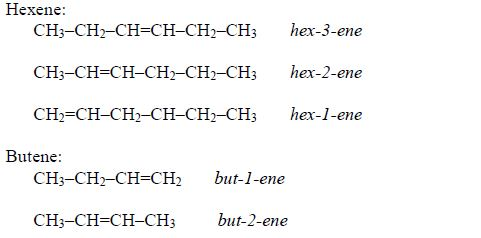
Alkynes
These are homologous series of hydrocarbons with the general formula CnH2n-2. The common structural feature is the presence of a carbon-carbon triple bond (CC). There is no member for n = 1 because, as for the alkenes, there needs to be at least two carbonatoms present to have a triple bond. Table 2.5 shows the first five members of the alkynes.
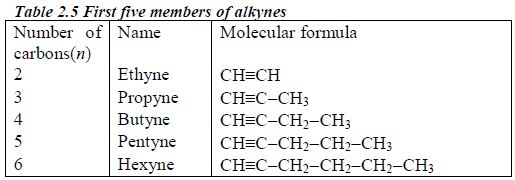
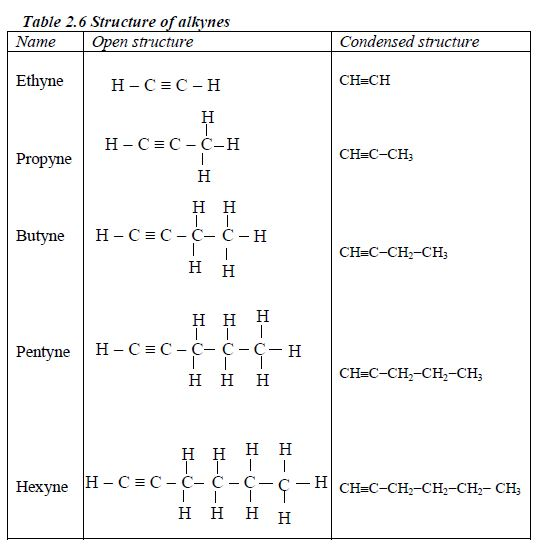
Nomenclature of alkynes
Rules for naming alkynes
- Alkynes have the ending –yne
- Triple bond is given the lowest number possible, usually lower than the functional groups.
- As for the alkenes the position of the triple bond must be included in the name.
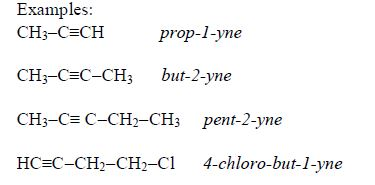
Isomerism
Alkynes show branching isomerism and positional isomerism. The alkyne with the molecular formula, C5H8, shows chain branching isomerism, for instance,
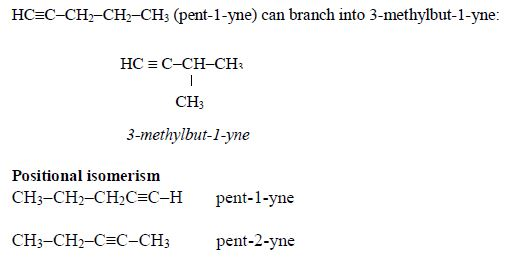
A General Formula to Identify the Families of Hydrocarbons
Apply a general formula to identify the families of hydrocarbons
Activity 1
Apply a general formula to identify the families of hydrocarbons
Properties of Hydrocarbons
The Physical Properties of Lower Hydrocarbons; Alkanes, Alkenes and Alkynes
Explain the physical properties of lower hydrocarbons; alkanes, alkenes and alkynes
Alkanes
- The first four alkanes are gases at room temperature, the next twelve (C5–C17) are liquids, and the rest are solids.
- The boiling and melting points of unbranched alkanes increase as the molar masses increase. The larger the molecule, the higher the boiling point or melting point.
- Branched alkanes have lower boiling points than the unbranched alkanes,
- Alkanes are very sparingly soluble in water but they easily dissolve in organic solvents.
- In an alkane molecule, each carbon atom forms four single covalent bonds. This means that alkanes are saturated hydrocarbons.
Alkenes
Here again we find that alkenes posses physical properties that arethe same as those of alkanes, i.e.
- They are insoluble in water but soluble in organic solvents suchas benzene, ether and chloroform.
- They are less dense than water.
- The boiling point increases with increase in molecular weighti.e., increase in the number of carbon atoms.
Alkynes
The physical properties of the alkynes are essentially the same as those of the alkanes and alkenes.
The Concept of Saturated and Unsaturated Hydrocarbons
Explain the concept of saturated and unsaturated hydrocarbons
Hydrocarbons are classified into two distinct categories: saturated and unsaturated. Saturated hydrocarbons contain only one carbon-carbon single bond. Unsaturated hydrocarbons contain at least one carbon-carbon double or triple bond. The unsaturated hydrocarbons are more reactive and they contain fewer hydrogen atoms bonded to the carbon atoms than saturated hydrocarbons.
The Chemical Properties of Alkanes, Alkenes and Alkynes
Compare the chemical properties of alkanes, alkenes and alkynes
Alkanes
Alkanes are not as reactive as other hydrocarbons. However, they exhibit the following chemical properties:
Combustion: alkanes burn in a sufficient amount of air to form carbon dioxide and steam.

Cracking: this is a process in which large alkanes are broken down into smaller hydrocarbons.

Halogenation: under special conditions (sunlight or 300°C), halogens react with alkanes in a substitution reaction. A substitution reaction is a reaction in which one atom or group of atoms replaces another.
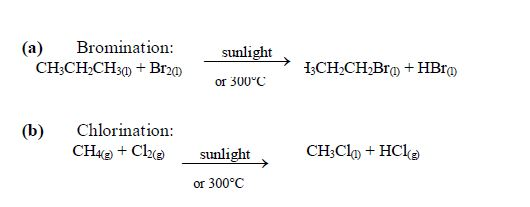
Alkenes
Alkenes are much more reactive than alkanes. This is because thedouble bond can break to form single bonds and add on otheratoms. Because the double bond allows them to add on moreatoms, alkenes are said to be unsaturated. The alkanes don‟t havedouble bonds and can‟t add on more atoms, so they are saturated.
Combustion: alkenes burn in sufficient supply of air (explodes)to form carbon dioxide and steam.

Addition reactions: Alkenes give a number of addition reactions in which two hydrogen atoms (or their equivalent) are taken intocombination per molecule to form a single product. The following are some of the addition reactions shown by alkenes:
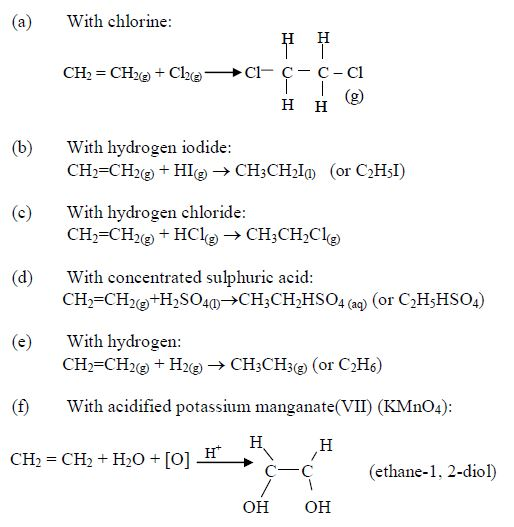
Alkynes
Addition: having carbon–carbon trip bonds, alkynes are unsaturated compounds. They give a number of addition reactions:
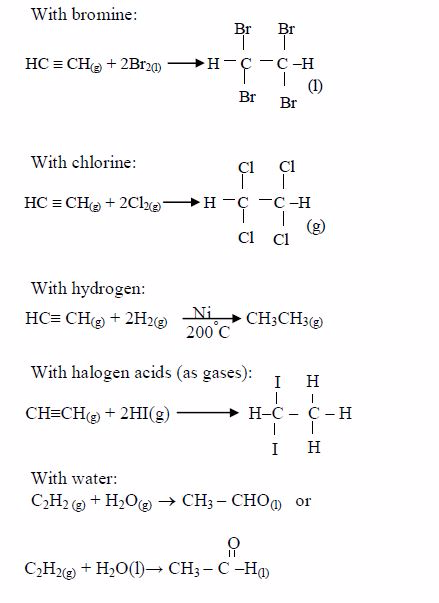
With acidified potassium manganate (VII) solution: At room temperature, with shaking, ethyne quickly decolourizes this solution (i.e. reduces it) with formation of ethanedioc acid
Polymerization

Combustion:

Salt formation:

This is a test for terminal alkynes, i.e. those with C≡C at the end. For those with C≡C at the centre, no reactions take place e.g. CH3–CH2–C≡C—CH2–CH3 + AgNO3→ No reaction!
Alcohols
Alcohols form a homologous series of general molecular formula, CnH2n+2, where n = 1, 2, 3, etc. for successive members of the group. The hydroxyl group, OH, is the characteristic functional group of the alcohols.
Preparation of Ethanol in the Laboratory
Prepare ethanol in the laboratory
Laboratory preparation of an alcohol involves heating a haloalkane with an aqueous alkali. Ethanol is prepared by heating a mixture of bromoethane and sodium hydroxide solution. The reaction proceeds as follows:

The ethanol, as indicated in the equation will be in solution. It canbe isolated by distillation.
Ethanol can also be prepared by fermentation of glucose wherebyyeast produces an enzyme called zymase which acts as an organiccatalyst to catalyse the degradation of glucose.
For substantial fermentation to take place, a mixture of glucose and yeast is left in a warm place for a considerable length of time.
The equation for the reaction taking place is:
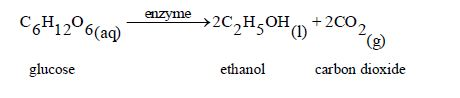
The Homology of Alcohols up to Five Carbon Atoms
Write the homology of alcohols up to five carbon atoms
Alcohols are named as if they are derived from alkanes by the replacement of hydrogen atom by the hydroxyl group (–OH). The lowest two members containing one and two carbon atoms in the molecule, are, respectively, methanol and ethanol. Their structural formulae are shown below:
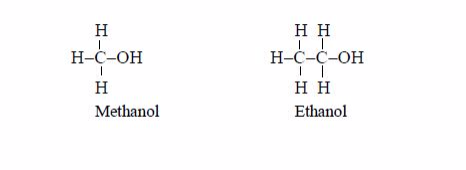
Other members of the series are as shown below:
Number of carbons per molecule (n) | Name | Formula |
1 | Methanol | CH3OH |
2 | Ethanol | C2H5OH |
3 | Propanol | C3H7OH |
4 | Butanol | C4H9OH |
5 | Pentanol | C5H11OH |
6 | Hexanol | C6H13OH |
7 | Heptanol | C7H15OH |
8 | Octanol | C8H17OH |
9 | Nonanol | C9H19OH |
10 | Decanol | C10H21OH |
The successive members of the series have molecular formulae which differ by CH2.
Like other series, alcohols exhibit isomerism. For instance, propanol (C3H7OH) can have two different structural formulae as exemplified below:
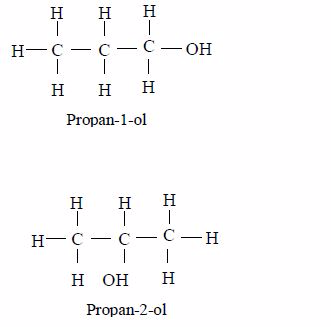
The first is called propan-1-ol because the OH group is on the first carbon atom.
The second is called propan-2-ol because the OH group is on the second carbon atom.
Structure of all Isomers of Saturated Alcohols up to Five Carbon Atoms
Write structure of all isomers of saturated alcohols up to five carbon atoms
Nomenclature of branched alcohols
Rules:
- For branched alcohols, the–OH group is given the lowest number than the alkyl group.
- The alcohol group (–OH) or the alkyl groups can be attached to different positions of the carbon chain. It is the different positions of these groups that result to the different names of the alcohol.
- The carbon chain may have several branches of different alkyl groups.
Examples:
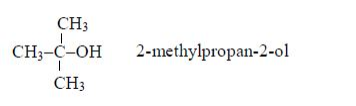
Note: Both the methyl (–CH3) and hydroxyl (–OH) groups are on carbon number 2. The longest unbranched carbon chain is
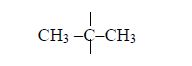
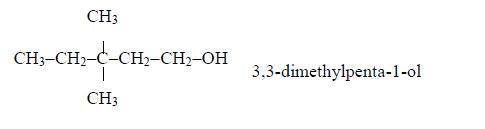
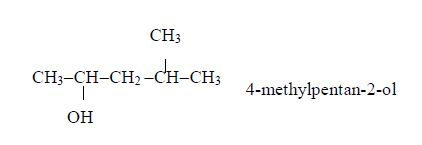
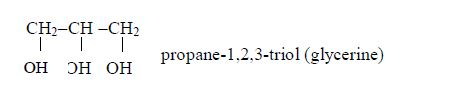
Isomers of Alcohols up to Five Carbon Atoms
Name all isomers of alcohols up to five carbon atoms
Alcohols exhibit chain branching isomerism as with all the other homologous series. In addition, they show an isomerism based on the position of the hydroxyl group (–OH) in the molecule.
Alcohols that have the formula CH3OH and CH3CH2OH have only one possible structure. They do not have isomers. The –OH group in ethanol, CH3CH2OH, is always on the last carbon atom.
With the formula CH3CH2CH2OH, however, the –OH group can either be attached to the first or second carbon of the carbon chain to give two isomers namely, CH3CH2CH2OH (propan-1-ol) and

Isomers of other members in the series are as follows:
Butanol: C4H9OH or CH3CH2CH2CH2OH
Isomers:
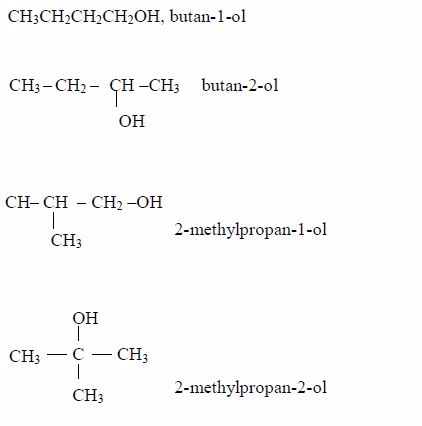
Pentanol: C5H11OH or CH3CH2CH2CH2CH2OH
ItsIsomers:
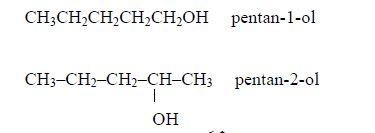
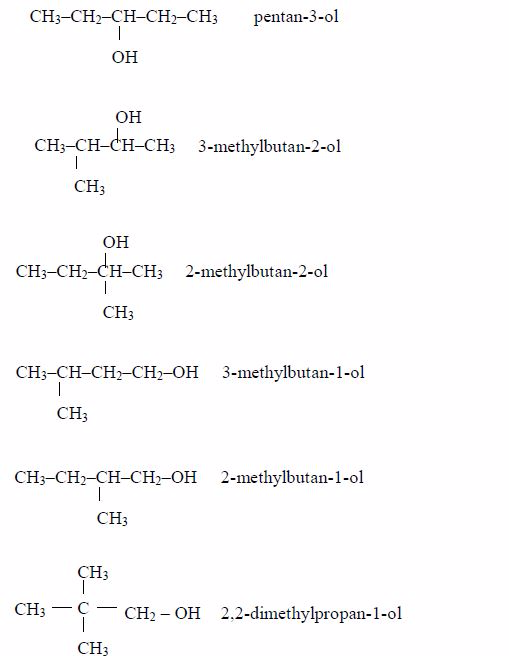
The Properties of Alcohol
Describe the properties of alcohol
Ethanol is the best known and most important of all alcohols. It has several different uses. Due to its diverse use, its name is used interchangeably with that of “alcohol” as if it represents all other classes of alcohols.
Physical properties of ethanol (alcohol)
- It is a clear, colourless liquid with a boiling point of 78°C.
- It is readily soluble in water and it mixes completely with it (miscible).
- It evaporates very easily when exposed to air (it is a very volatile liquid).
Chemical properties of ethanol
Reaction with oxygen (combustion)
It burns well in oxygen giving carbon dioxide and water, and plenty of heat.

This reaction is the basis of its use as a fuel.
Reaction with sodium metal
Ethanol reacts vigorously with sodium to produce sodium ethoxide and hydrogen. However, the reaction is not as vigorous as the reaction between sodium and water.

Methanol reacts similarly to produce sodium methoxide and hydrogen:

Reaction with ethanoic acid (esterification)
Alcohols react with organic acids to form sweet-smelling oily liquids known as esters.

The symbol, H+, indicates that the reaction takes place in acidified conditions. Normally dilute sulphuric acid is added as a catalyst for this esterification reaction.
Reaction with concentrated sulphuric acid
Concentrated sulphuric acid dehydrates ethanol to give ethene. The reaction takes place at 180°C.

Reaction with phosphorus pentachloride
Ethanol reacts vigorously with phosphorus pentachloride to give chloroethane, phosphorus oxychloride and hydrogen chloride.

Hydrogen chloride is evolved as white fumes, and this reaction is used to test for alcohols and other hydroxyl compounds.
Oxidation
Ethanol is oxidized by strong oxidizing agents such as warm acidified potassium dichromate or acidified potassium permanganate to ethanoic acid.

The Uses of Alcohol
Explain the uses of alcohol
Alcohol is used as an alcoholic beverage. Different alcohol drinks contain different amounts of ethanol. Beer and wines contain 8–13% of ethanol. Sprits such as whisky, gin, brandy, rum, etc. contain about 35–40% of ethanol. However, these beverages must only be drunk with moderation as too much consumption of alcohol can lead to heath problems.
Ethanol as fuel.
- Ethanol burns with a clear flame, giving out quite a lot of heat.C2H5OH(l) + 3O2(g) → 2CO2(g) + 3H2O(g) + heat On a small scale, ethanol can be used as methylated spirit (ethanol mixed with methanol or other compounds) in spirit lamps and stoves.
- Ethanol produced by fermentation of sugar from sugar cane has been used as an alternative fuel to gasoline (petrol), or mixed with gasoline to produce “gasohol”.
Ethanol as a solvent.
Alcohol is a good solvent, dissolving many compounds that are insoluble in water. The fact that it evaporates easily makes it a good solvent in products like glues, paints, varnishes, deodorants (perfumes), and aftershaves.
It is used in the manufacture of many chemicals
For example, it is used in making sweet–smelling liquids called esters which are used as solvents, in food flavourings, and as fragrance in beauty products (cosmetics).
Ethanol is also used as an ingredient in iodine tincture (a mixture of alcohol and iodine) which is largely used in hospitals for treatment of wounds.
The Harmful Effects of Alcohol
Explain the harmful effects of alcohols
In spite of its widest use as a useful product, ethanol, taken as an alcoholic beverage, has a lot of detrimental health effects. Some of the effects of alcohol are as follows:
- Even just one drink impairs coordination and judgement. It is a factor among the many causes of road accident as it leads to blurred vision.
- It leads to lack of muscular control (e.g. drunken stagger) and ultimately to coma, the state in which a person is said to be dead–drunk.
- Prolonged consumption of too much alcohol causes liver deterioration (cirrhosis of the liver) which can cause liver failure and death. Also heavy drinking eventually damages the muscle tissue of the heart. There may well be some long–term damage to the brain. All these health effects accelerate death.
- Alcohol is a depressive drug and can be addictive. Occasional drinking may lead to alcohol addiction, a condition during which a person is said to be alcoholic. When a drunkard finds him/herself in such a condition it is very difficult to go without drinking. This can eventually lead to poverty as a drunkard spends most of his/her time and money drinking (buzzing).
- Alcohol can make someone aggressive. This accounts for many arrests, conviction and jail sentences.
- Excessive drinking causes depression and other mental disorders.
- It can lead to gastric ulcers, high blood pressure and cancer of the mouth, throat, and gullet. People who smoke as well are at greater risk from thesecancers.
Women are at greater risk than men from the harmful effects of alcohol. One reason is that men‟s bodies have higher water content. So alcohol in their body fluids is more diluted.
Drinking during pregnancy can damage the baby‟s brain and heart, and slow down its growth so that it is born underweight.
Carboxylic Acids
Carboxylic acids form a homologous series of the general formula CnH2n+1COOH (or CnH2n+1CO2H), where n = 1, 2, 4, etc. for successive members of the group. All these acids have the characteristic functional (carboxyl) group, –COOH, attached to a hydrocarbon chain.
Natural Sources of Organic Acids
Identify natural sources of organic acids
There are various natural sources of organic acids. Some of these sources are:
- milk (lactic acid)
- citrus fruits (citric acid);
- tobacco (nicotinic acid); and
- tea (tartaric acid).
The Oxidation of Ethanol to Ethanoic Acid
Explain the oxidation of ethanol to ethanoic acid
When exposed to open air, ethanol is oxidized (by oxygen of the air) to ethanoic acid. The reaction for the process occurs thus:

The Structures of the Homologues of Carboxylic Acids up to Five Carbon Atoms
Write the structures of the homologues of carboxylic acids up to five carbon atoms
Carboxylic acids are named as if they are derived from alkanes by the replacement of one hydrogen atom by the –COOH group. The two lowest members, containing one atom and two carbon atoms respectively, are:
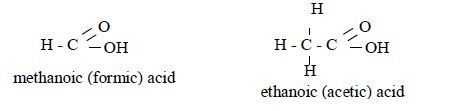
The other members of the homologous series are as shown below:
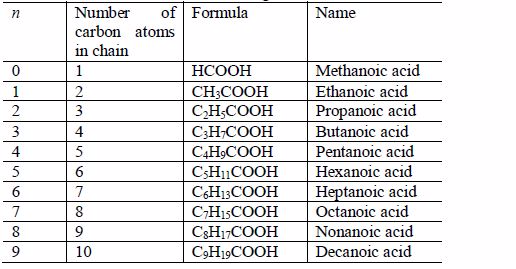
The successive members of the series have molecular formulae which differ by –CH2 It is important to remember that every carboxylic acid molecule contains the functional group –COOH which is called the carboxyl group.
The Isomers of Carboxylic Acids up to Five Carbon Atoms
Name the isomers of carboxylic acids up to five carbon atoms
Like other organic compounds, carboxylic acids also exhibit isomerism. Isomers of carboxylic acid are a result of branching of the hydrocarbon end (R) rather than the position of the carboxyl group in a molecule of the carboxylic acid. More isomers of the carboxylic acids can be created by branching the hydrocarbon end in as many different ways as possible.
Rules
The carbon of the carboxyl group (–COOH) is considered as carbon atom number 1.
Identify the positions of the alkyl group(s) attached to the (longest) acid chain. For example, in a molecule,
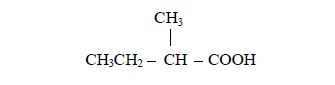
the alkyl group is methyl (–CH3) and it is attached to carbon number 2.
Name the branched alkyl group, followed by the name of the acid to which the alkyl group is attached. For example, in the case above (rule no.2):
- the alkyl group is methyl;
- it is attached to carbon number and
- the acid to which it is attached is butanoic acid,CH3CH2CH2COOH
Therefore, the name of the compound is 2-methylbutanoic acid.
In case there occurs more than one alkyl groups in the compound the prefixes di(2), tri(3), tetra(4) etc (as it was the case in alkanes) may be used. For, example in the compound
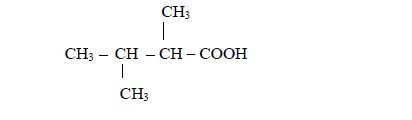
- there are two methyl groups, one attached to carbon number 2 and the other to carbon number 3; and
- they are both attached to butanoic acid chain.
Therefore, the name of the compound is 2,3-dimethylbutanoic acid.
Isomerism and nomenclature
Branching isomerism is found in this homologous series. Isomerism in carboxylic acids begins from butanoic acid, C3H7COOH. The first three members of the series do not show isomerism because their hydrocarbon ends do not form branches. The following are the structures and names of the isomers of carboxylic acids up to five carbon atoms:
- Butanoic acid, C3H7COOH or C3H7CO2H or CH3CH2CH2COOH
Isomers:
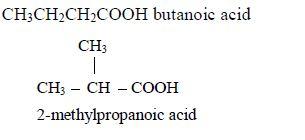
- Pentanoic acid, C4H9COOH
Isomers
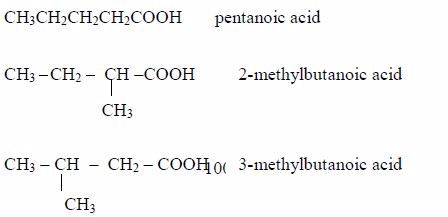
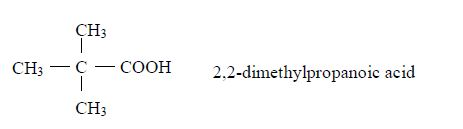
The Properties of Carboxylic Acids
Explain the properties of carboxylic acids
Carboxylic acids are weak acids. They are slightly ionized in dilute solutions.

Like inorganic acids, their solutions contain H+ ions. The presence of H+ ions give the solutions acidic behaviour, that is, their solutions affect indicators, just like the inorganic acids do.
Neutralization
Like inorganic acids, carboxylic acids react with metals, alkalis, carbonates, and hydrogen carbonates to form salts. For example:
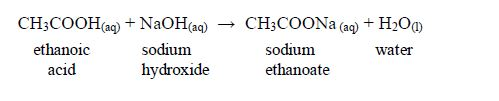
Esterification
The reaction between carboxylic acids and alcohols is called esterification. The acids will react reversibly with alcohols to form sweet–smelling esters. Concentrated sulphuric acid is a catalyst for the reaction.

The reaction can be reversed to recover an acid and alcohol again by boiling the products (an ester + water) with a mineral acid (HCl or H2SO4) or with an aqueous alkali (KOH or NaOH) as a catalyst.
Esters are manufactured for use as solvents, food flavourings, and fragrance for perfumes and beauty products. Ethyl ethanoate is just one example of many esters. The esters usually have strong and pleasant smells. Many of these compounds occur naturally. They are responsible for the flavours in fruits and for the scents of flowers. Fats and oils are naturally occurring esters used for energy storage in plants and animals. Some of the naturally occurring esters include:
- vegetable oils e.g. palm oil, groundnut oil, cashewnut oil, olive oil, sunflower oil, etc; and
- animal fats.
All esters contain the functional group,
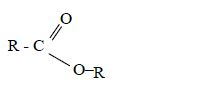
, where R is any alkyl group.
Preparation of Soap from Animal Fats or Vegetable Oil
Prepare soap from animal fats or vegetable oil
Vegetable oils are formed from fatty acids and an alcohol called glycerol (also called glycerine). Fatty acids are carboxylic acids with long chains of carbon atoms. They are called “fatty” because the long chains repel water, making them immiscible with water. Glycerol or glycerine (or propane–1,2,3-triol) has three –OH groups. This is how fatty acids and glycerol react:
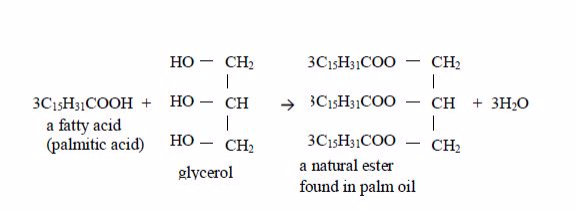
Preparation of soap from oils
Soap is made by heating animal fats or vegetable oils with sodium hydroxide solution. The oils react with the solution of sodium hydroxide and break down to form glycerol and the sodium salts of their fatty acids. These salts are used as soap. The reaction equation is:
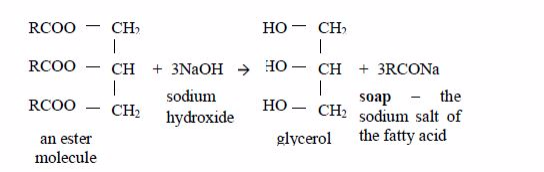
This process is known as saponification. The soap you buy is made from a blend of different oils. When soap dissolves in water it ionizes thus:

The cleansing agent in soap is the ion, RCOO–
Topic three
Soil Formation
Soil Formation
Soil Formation
Describe soil formation
Soil is formed by the process of weathering. All types of weathering (physical, chemical or biological) result to disintegration of rocks into smaller particles. Air and water enter the space between these particles and chemical changes take place, which lead to the production of chemical substances. Bacteria and plant life soon appear. When plants and animals die, they decay and produce humus. Bacteria and other decomposers play a vital role in the decomposition of plant and animal substrata. The end product of these mechanical, chemical and biological processes is soil.
Therefore, soil can be defined as unconsolidated mineral (inorganic) and organic material on the immediate surface of the earth‟s crust that serves as the medium for plant growth.
All soils contain mineral matter, organic matter, water, air and living organisms, especially bacteria. If any one of these is substantially reduced in amount or is removed from the soil, then the soil deteriorates. There are many types of soil and each has specific characteristics related to the climate, the vegetation and the rock of the region in which it forms. The weathering processes of a region also play an important part in determining soil characteristics. The relationship of these factors is as shown in figure 3.1.
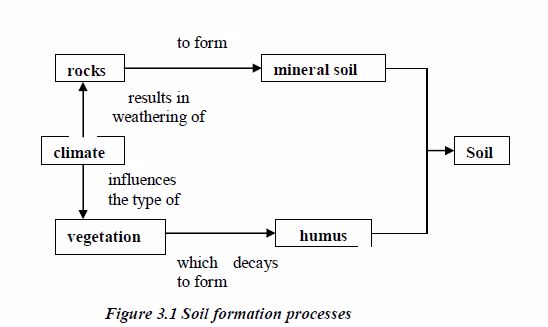
The Factors Influencing Soil Formation
Describe the factors influencing soil formation
Information about soil formation can lead to better soilclassification and more accurate interpretation of soil properties.There are several factors responsible for soil formation. Thefactors include climate, living organisms, relief (topography),parent material and temperature. All the factors, except time,depend to a greater or lesser extent upon each other, upon the soilitself or upon some other factor. None of the factors can beconsidered more important than any other, but locally one factormay exert a particular strong influence. These factors areexplained in details below.
Parent material
Parent materials are made up of mineral material or organic matter or a mixture of both. The organic matter is usually composed predominantly of unconsolidated, dead and decaying plant remains. The mineral material, which is the most widespread type of parent material, contains a large number of different rock– to form Climate which decays to form results in weathering of influences the type of climate rocks vegetation humus Climate Soil mineral soil Climate forming minerals and can be in either consolidated or unconsolidated state.
Some rocks are more easily weathered than others. Acidic rocks are more resistant to weathering than basic rocks. The parent rock affects soil texture and water permeability.
Parent rock with fine particles is more resistant to chemical weathering than mechanical weathering. Very compact parent rocks like sandstone are very much resistant to weathering. Porous rocks weather easily by chemical processes. This is because they have large surface areas for weathering agents to act upon.
Climate
Climate is the principal factor governing the rate and type of soil formation as well as being the main agent determining the distribution of vegetation. The dead vegetations decay to form humus as one of the components of the soil.
To understand well the influence of climate on soil formation let us have a look at its components and how each of these components affects soil formation.
Temperature
The main effect of temperature on soil is to influence the rate of reactions; for every 10°C rise in temperature, the speed of a chemical reaction increases by a factor of 2 or 3 (twice or thrice). Temperature, therefore, influences the speed of disintegration and decomposition of the parent materials and its consolidation to form the soil.
Rainfall (water)
The water in soils includes all forms of water that enter the soil system and is derived mainly from precipitation as rain. The water entering soils contains appreciable amounts of dissolved carbodioxide, forming a weak carbonic acid. This dilute, weak acid solution is more reactive than pure water. It thus reacts with unconsolidated minerals and organic matter, breaking them down into mineral (clay, sand) and organic debris (humus) respectively.
Organisms
The organisms influencing the development of soils range from microscopic bacteria to large mammals including man. In fact, nearly every organism which lives on the surface of the earth or in the soil affects the development of soils in one way or another. More important soil organisms of interest to soil formation are as follows:
Higher plants.
Higher plants (particularly grasses) extend their roots into the soil and act as binders. So they prevent soil erosion. The roots also assist in binding together small groups of particles hence developing a crumby or granular structure. Large roots are agents of physical weathering as they open and widen cracks in rocks and stones. When plants die they contribute organic matter to the soil, which acts as a binder of the soil particles. Higher plants intercept rain and they shelter the soil from the impact of raindrops. They also shade the soil and hence reduce evaporation.
Vertebrates
Mammals such as moles, ground squirrels and mice burrow deeply into the soil and cause considerable mixing up of the soil, often by bringing up subsoil to the surface, and creating burrows through which the top soil can fall and accumulate within the subsoil.
Microogarnisms
These include bacteria, fungi, actinomycetes, algae and protozoa. These organisms act as decomposers of organic and even mineral matter.
Mesofauna
These include earthworms, nematodes, millipedes, centipedes and many insects, particularly termites and ants. Activities of mesofauna include:
- ingesting organic mineral materials e.g. earthworms and millipedes;
- transportation of materials e.g. earthworms, millipedes, termites, beetles, etc; and
- improvement of soil structure and aeration.
Man
Activities of man are too many and too diverse. Man‟s roles include:
- Cultivation of soils for production of food and tree crops, which in many cases has negative effects causing impoverishment of the soil and erosion.
- Indiscrimate grazing, casual burning, cutting of trees, manure and fertilizer use, all of which alter the soil characteristics.
Relief (Topography)
This refers to the outline of the earth‟s surface. All land surfaces are constantly changing through weathering and erosion. It may take millions of years, in the case of Himalayas and the Andes, to be worn down to flat undulating surfaces. The soils on steep mountain slopes are shallow and often stony and contain many primary minerals. In areas where the difference in elevation between the highest and the lowest point is great, then climatic changes are introduced. These differences in elevation, slope, slope direction, moisture and soil characteristics lead to the formation of a number of interesting soil sequences.
Time
Soil formation is a very slow process requiring thousands and even millions of years. Hence, it is impossible to make definite statements about the various stages in the development of soils.This is because it takes a considerable period of time for a particular soil type to be formed and categorized.
Soil Reaction
The Concept of Soil Reaction
Explain the concept of soil reaction
Soil reaction refers to how acidic or alkaline a soil is. It isexpressed as a pH value. The soil can be acidic, neutral or alkaline. Extremely acidic soils can have pH values below 4.5 and on the other hand, very alkaline soils can have pH values up to,and even higher than 9.0.
Nearly all soils have pH values between 4 and 8. Soils with pH< 4 generally contain sulphuric (IV) acid, while those with pH <8 contain a high percentage of Na+ ions and thus they are alkaline.
pH is defined as the negative logarithm (to base 10) of the hydrogen ion activity (concentration):
pH = -log10[H+] where [H+] denotes the concentration of H+ ionsin grams/litre. This is the same as saying that pH is the logarithmof the reciprocal of [H+]:
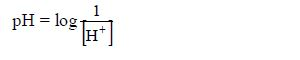
The greater the [H+], the lower the pH and the more acidic the soilis. Acidic soils are common in humid regions, particularly thetropics, where rainfall is sufficiently high to leach theexchangeable bases from the top soil. Alkaline soils, on the otherhand, are characteristic of the arid regions of the world where,because of low rainfall, there is a high concentration of basiccations (Ca2+, Mg2+, Na+, etc) in the surface soil layer. Generally,acidic soils occupy a large area of arable land than alkaline soilsdo. Because of this, acidic soils are considered to be moreimportant, at the practical level, as compared to alkaline soils.
Causes of soil acidity
Having known that the soil can be acidic and that the acidity is aresult of great concentration of H+ ions in the soil, let us knowlook at the causes of soil acidity. The causes of soil acidity includethe following:
- Leaching: Heavy rains may leach bases like Ca2+, Mg2+, K+ andNa+ from the soil to the ground water table, leaving a surplus ofH+(aq) in the soil.
- Soil Microorganisms and root respiration produce carbondioxide which forms weak carbonic acid with the soil solution.
- Near industrial regions, acid rain (often pH 2–4) may bringsulphuric (IV) acid and nitric (V) acid to the soil.
- Acid mineral fertilizers, like ammonium sulphate (VI) andammonium chloride make the soil solution more acidic due to oxidation and hydrolysis:
Oxidation:

Hydrolysis:

Also the NH4OH produced by oxidation and hydrolysis dissociates further to give NH4+ and OH– ions. The NH4+ ions produced undergoes microbial and enzymatic oxidation (as show above) to release more H+ ions to the soil.
5. Nitrification of ammonium ions by bacteria produces H+(aq):

6. Organic acids produced during the decomposition of organic matter also contribute to soil acidity. Due to such reasons, most soils in the humid tropics are acidic.
7. The Al3+ ions present in soil solution contribute to soil acidity indirectly when they are hydrolysed:

For simplicity and easy understanding, the equation for hydrolysis of Al3+ ions is sometimes represented in a single equation as:

8. Small amounts of ions such as NO3‾, NO2‾, H2PO4‾, SO42‾and Cl‾ present in the soil solution also contribute to soil acidity.
The above causes to soil acidity can be categorized as either natural or artificial. The artificial causes are acid rain and acid mineral fertilizers which are a result of deliberate human actions. The rest of the causes are classified natural because they occur naturally.
The pH of a Given Soil Sample
Measure the pH of a given soil sample
The pH of a soil can be tested by using Soil pH Kit. The kitcomprises of equipment and dyes (pigments) that are employed insoil pH determination.
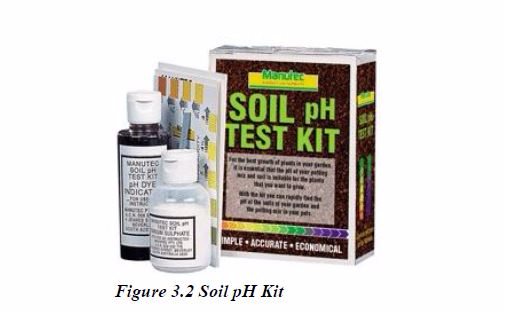
Soil pH kit is simple to use and can be used many times. Colour indicator dye and chart easily helps to find out pH. Knowledge of soil pH is very important to a farmer. Most plants grow well in soils with pH ranging between 5.5 and 7.5.
The following is a quick and accurate way to find soil pH using a soil pH kit:
1. Getting prepared
First collect your equipment
You will need a clean trowel and a clean container for each sample you take. You can use clean boxes, plastic bags, or any convenient container to collect your sample. For powder-based kits you will need distilled water for the test itself.
Decide where to take the samples
Soil pH can vary in different parts of your garden either naturally or through different types and levels of cultivation. You may be able to see clear differences in colour, texture and humus content. Therefore, aim to take a number of samples from different areas and test each one separately.
2. Collecting the soil samples
For each area you are sampling, scrape away the top soil to a depth of about 5 cm. This prevents the reading being affected by any top dressings or mulches you have applied or any accumulation of leaf litter or pine needles.
Now break up the soil to a depth of about 12 cm and take the sample from the bottom level. Collect more than you think you will need as you will be picking out all the lumps, stones, twigs etc. Make sure you label each sample so that you know where they have come from.
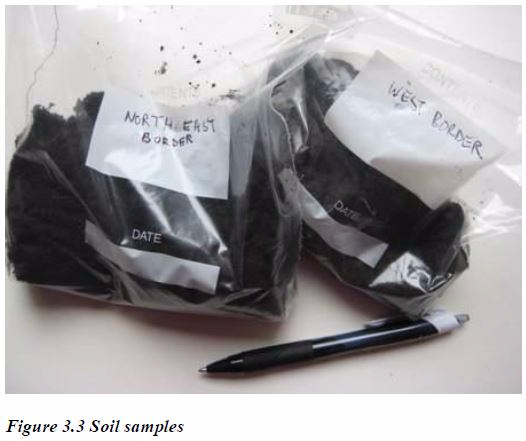
3. Sample preparation
- Pick out any stones, roots and twigs and leave the sample to dry.
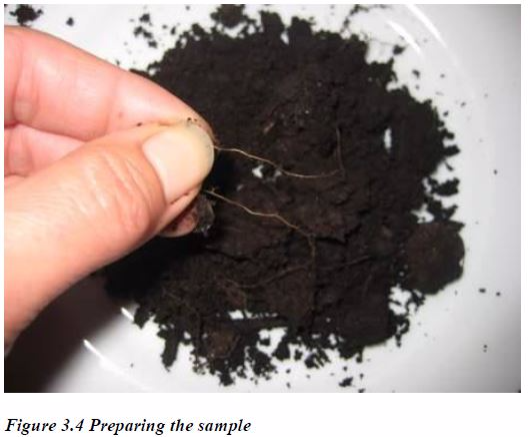
- Break up the dry sample with the back of a clean teaspoon or the tip of a clean trowel, and place the specified amount in the test tube or test chamber provided.
4. Carry out the pH test
This stage will vary depending on the type of kit you have purchased.Before going any further, it is important to note that soil pH test is purely a qualitative test. In this test, barium sulphate is often used as a reagent.
Use of barium sulphate
Barium sulphate is used in soil testing as a flocculant. It causes the fine soil particles to clump together and sink, leaving a clear test solution. This enables you to make an accurate colour comparison. If you have a clay soil with lots of fine particles you may need to add extra barium sulphate to clear the liquid. This is another advantage of liquid-based tests where the barium sulphate powder is provided separately.
Liquid-based kits
If your kit contains a liquid test solution, you will usually have to add a scoop of the provided barium sulphate powder to the sample followed by the specified amount of test solution. If you have a clay-based soil it is useful to add extra barium sulphate right at the start. Put the cap on the test tube and shake it well. Leave it to settle for the required time, normally 10 minutes.
Powder-based kits
For kits containing the reagent in powder form you will have to add the specified quantity of powder (this usually contains the appropriate amount of barium sulphate as well as the reagent) followed by the required amount of distilled water. Put the cap on the test tube and shake well to mix. Allow to settle for the specified time, usually around 10 minutes.
5. Read the results
Compare the resulting colour of the solution against the supplied colour chart. Don't leave the solution for much longer than the stated time because the colour may start to change and you won't get an accurate result. Try to do this in good natural light but away from bright sunlight to make an accurate comparison.The following are the results obtained from two soil samples tested: The sample from the West Border (above) is neutral (it is neither acidic nor basic).
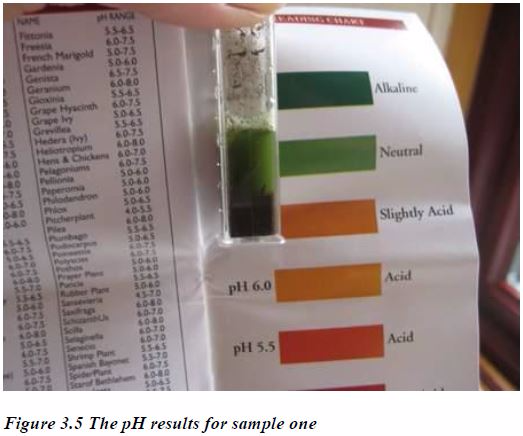
The soil sample from the North-east border, however, was found to be acidic, with pH 6.0. The soil contains a lot of organic matter, such as garden compost and rotten manure, which tends to lower soil pH, making it more acidic.
However, both soils fall well within the pH range (5.5 to 7.5) acceptable to most plants so the farmer can just carry on with farming as before. There is no need for soil pH amelioration.
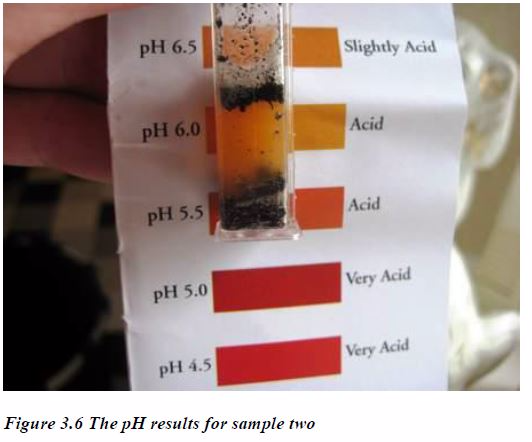
Determination of the soil pH is important because it helps to identify the pH of different soils and hence making the correct decision on different kinds of crops that can be grown on a soil with a particular pH.
Plant growth is affected by the acidity or alkalinity (pH) of the soil. Soils with high peat content, or with minerals such as iron compounds, or with rotting vegetation and lack of oxygen, tend to be acidic. Their pH can reach as low as pH 4. Soils in limestone orchalky areas are alkaline (up to pH 8.3). Different plants prefer different pH conditions. Farmers and gardeners can test the soil pH to see whether it suits the needs of particular plants. An example of preferred soil pH conditions for different crops is given below:
Crop | Preferred pH |
Irish potato | 4.5 – 6.0 |
Chicory, parsley | 5.0 – 6.5 |
Carrot, sweet potato | 5.5 – 6.5 |
Cauliflower, garlic, tomato | 5.5 – 7.5 |
Broad bean, onion, cabbage and many others | 6.0 – 7.5 |
Managing the Soil pH by Using Different Liming Materials
Manage the soil pH by using different liming materials
If the soil is strongly acidic (pH < 5), most crops will give only very poor yields if any. In such a soil, the acidic H+(aq) and Al3+(aq) ions prevail while the basic nutrient ions such as Na+, Mg2+. Ca2+, etc are not sufficiently available.
In order to raise the pH of such a soil, basic compounds of calcium and magnesium are added and mixed well with the top soil; e.g. the oxides, hydroxides, carbonates and silicates of calcium and magnesium, commonly called agricultural limes. All these compounds have the effect of neutralizing the acidity of the soil. If the soil is too alkaline, it helps to dig
When lime is added to an acidic soil, the liming material usually reacts with the water and/or with the carbonic acid in the soil and dissolves e.g:
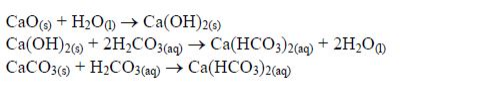
All liming materials, directly or after dissolution, react with hydrogen ions (H+) adsorbed on the soil colloids e.g:
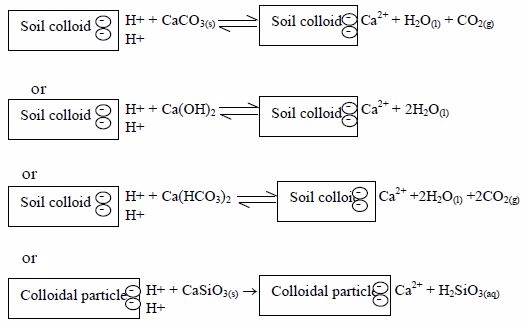
Thus, by liming an acid soil, the percentage base saturation and the pH of the soil is raised and carbon dioxide is produced. The amount of lime needed depends on the pH of the soil, its texture, structure and its content of organic matter.
Overliming, that is, raising the soil pH to above 7.5, is a danger especially in soils of low cation exchange capacity and hence low reserve acidity. It reduces the availability of P, K, Fe, Mn, B and Zn often so much that crops suffer from the deficiencies of these nutrients. Often, crops become stunted and turn yellow. Therefore, overliming must be avoided by all means.
Plant Nutrients in The Soil
The Essential Plant Nutrients
Categorize the essential plant nutrients
MACRO AND MICRONUTRIENTS
Macronutrients are those mineral nutrients that are required by plants in greater amounts. They constitute about 99% of plants‟ requirements.
Macronutrients, also referred to as major nutrients are further divided into primary and secondary macronutrients. Primary macronutrients are the elements that are required by plants in relatively large quantities (60% of the plant‟s requirements). They are nitrogen, phosphorus and potassium. The secondary macronutrients are calcium, magnesium and sulphur. These elements contribute the remaining 39% of the plant‟s needs
Micronutrients, also referred to as trace elements, are those mineral nutrients that are required by plants in smaller amounts. They constitute about 1% of plants‟ requirements.
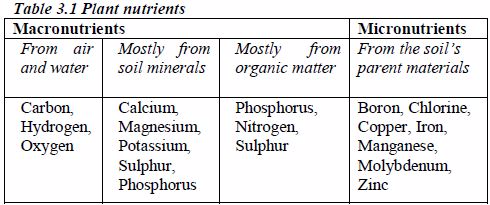
Plants use the sugars for energy supply and to produce cellulose (for the cell walls) and starch (for storage). Proteins are made from sugar and nitrogen. They are used to synthesize protoplasm which is vital for the plant cells.
INTAKE OF NUTRIENTS BY PLANTS
Except for some gaseous carbon dioxide, oxygen and sulphur dioxide, all nutrients enter the plants in the form of ions usually from the soil solution through the roots.
The metals are absorbed by the plant roots in the form of their cations. Nitrogen is taken in as ammonium or nitrate (V) ions; phosphorus as dihydrogenphosphate (V) ions; sulphur as sulphate (VI) ions and chlorine as chloride ions.
The nutrient ions move towards the plant roots either with the flow of the soil solution (since the roots also take in water) or by diffusion. The ions diffuse from areas of high concentration to those of low concentration caused by the intake by the roots.
Plant roots either absorb equal numbers of positive and negative charges in the form of ions from the soil solution or they exchange one ion against another one with the same charge, usually H+ or OH–. Thus, roots possess electrical charges on their surfaces which can hold and exchange ions.
The Functions of Each of the Primary Macronutrients in Plant Growth
Explain the functions of each of the primary macronutrients in plant growth
AVAILABILITY, FUNCTION AND DEFICIENCY OF PLANT NUTRIENTS
All essential plant nutrients perform specific functions to aid plant growth or reproduction. They must all be available in the right proportion to facilitate optimum plant growth.
Carbon, Hydrogen and Oxygen
Availability: carbon and oxygen are taken in by plants through the stomata in the form of carbon dioxide from the air. Thus, they are never in scarce supply. Hydrogen (and oxygen) is supplied by water
Use in the plant: in the presence of sunlight, the green parts of the plant synthesize carbohydrates (sugars) from carbon dioxide and water.
Deficiency symptoms: plants wilt and die if they do not obtain sufficient water.
Nitrogen
Availability: only ammonium and nitrate (V) ions are available to plants. Usually about 98% of a soil‟s nitrogen is available (in organic matter). Organic matter decomposes by microbial action to form NH4+. This process is called ammonification. Almost any microbe can carry out ammonification.
There is another process called nitrification, which is carried out only by a few specific bacteria e.g. nitrosomanas. Nitrosomanas bacteria oxidize ammonium ions to nitrate (V) ions:

A few living organisms can fix nitrogen from the air. The best known are rhizobia (legume bacteria) and the free living bacteria such as azotobacter and clostridium, also the blue-green algae.
Plant needs: plants need more nitrogen than any other nutrient. However, their needs vary greatly: plants with high vegetative growth (stems, leaves, etc) have high N needs (maize, sorghum, rice, sugar cane, pasture grasses, most vegetables). Root crops (cassava, sweet potatoes, taro) have lower N needs. Legumes (alfalfa, desmodium, kudzu, all types of beans and peas, ground nuts, etc) may not need N fertilization if the proper strain of rhizobia is fixing N for them.
Use in the plant
- It is used to build amino acids, nucleic acids, many enzymes, chlorophyll, generally speaking: all proteins.
- It promotes the vegetative growth in plants. It is therefore important in the growth of plants in which leaves are harvested, such tobacco and vegetables.
- It is an essential element in cell division. It is therefore needed for plant growth.
- It increases grain size and protein content in cereals.
- It promotes root growth.
Deficiency symptoms: leaves turn yellow and finally die, because chlorophyll can not be build up. Then due to lack of chlorophyll the plant grows slowly, because the chlorophyll is needed for carbohydrate production. The shortage of chlorophyll is called chlorosis.
Excess nitrogen: excess nitrogen causes dark green succulent vegetation with weak stems, often at the expense of seed or fruit production, e.g. in grain crops, in tomatoes and beans.
It causes the potatoes to be watery. It delays crop maturity, and makes plants more vulnerable to attack by diseases and pests. Thus, fertilizers must be carefully dosed.
Available N is easily leached since NH4+ is rapidly nitrified in a warm climate, and NO3– is not adsorbed by soil colloids. Thus, it is important to apply fertilizer at the right time in order to avoid leaching.
Phosphorus
Availability: only H2PO4– and to a lesser extent HPO42– are available to plants.
Plant needs: plants need less phosphorus than nitrogen or potassium.
Use in plant
- Phosphorus is an essential component of the genetic material of the cell nucleus (RNA, DNA); also in ADP and ATP, which play a vital role in photosynthesis, amino acid and fat metabolism, etc.
- It increases the grain yield e.g. of millet, sorghum and rice because it promotes the formation of tillers.
- It promotes root growth.
- It strengthens the resistance of plants to diseases.
- Also rhizobia bacteria need it in order to fix nitrogen from the air.
- It hastens plant maturity.
Deficiency symptoms
- Dark green colouration
- Purple spots or streaks.
- Stunting, delayed maturity.
Fertilizers: superphosphates, triple phosphates, ammonium phosphates.
Potassium
Availability: only the K+ ions of the soil solution are available to plants. Hydrated potassium ions attached to soil colloids are readily available because they are not bonded strongly to the surface of the colloids.
After nitrogen, potassium is the second-most element needed by plants. Starch and sugar crops (cassava, sweet potatoes, banana, sugarcane) have relatively high needs of K.
Use in plants. Potassium is present in plants in the form of it ions only. It does not form any integral part of the structure of any known organic compounds in plants.
- Potassium is an activator of a number of enzymes involved amino acid synthesis and several enzymes concerned with carbohydrate and nucleic acid metabolism.
- Potassium aids in the uptake of other nutrients and in their movements within the plant e.g. potassium ions and nitrate (V) ions may move together.
- Potassium is also important in the metabolism of carbohydrates and translocation of food. Thus, it promotes starch and sugar formation.
- It regulates osmosis in cells, improves tissue formation and assists in protein synthesis.
- It strengthens plant stalk, hence preventing lodging and microbial attack.
Deficiency symptoms.
- Stunting: First the edges of the older leaves and then areas between veins turn yellow and finally brown. Small, brown necrotic spots develop while the veins are still green.
- Leaf curling and premature leaf fall.
Fertilizers: potassium chloride, potassium sulphate, potassium nitrate. Wood ashes and their aqueous extract (potash) contain potassium carbonate. Tobacco stems contain about 5% potassium, and cocoa shell meal about 3%.
Calcium
Availability: only Ca2+ ions in the soil solution are available to plants. Calcium comes from CaCO3 (calcite), gypsum (CaSO4·2H2O), apatite and other minerals.
Use in plants.
- Calcium is a constituent of cell walls and hence makes the straw stiff and resistant to lodging.
- It is essential for cell division.
- It promotes early root and seed development.
- It regulates the intake of potassium by plants.
- It neutralizes harmful organic acids like ethanedioic (oxalic) acid in plants, thus detoxifying them:
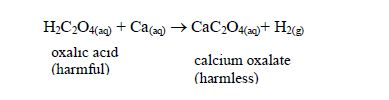
Magnesium
Availability: plants take in Mg2+(aq) through roots and leaves.
Use in plant;
- Magnesium is vital to the production of chlorophyll, because every molecule of chlorophyll contains a magnesium ion at the core of its complex structure. Most of the magnesium in plants is found in either chlorophyll or seeds. A lesser part is distributed in other parts.
- Aids in the translocation of carbohydrates.
- Regulates the uptake of other nutrients.
- Part of the distributed magnesium functions in the enzyme system involved in carbohydrate metabolism.
Fertilizers: Mostly dolomitic limestone (CaCO3·MgCO3). The principal magnesium fertilizer is magnesium sulphate (VI), sometimes known as epsom salt. It is soluble in water and can be sprayed onto the leaves.
Sulphur
Availability: Plants take in sulphate (VI) ions (SO42–) from the soil solution and sulphur dioxide from the air.
Its Use in plants.
- Sulphur is a vital part of plant proteins since cystine and methionine are sulphur-containing amino acids.
- Sulphur is also essential for the action of enzymes involved in nitrate (V) production.
The Deficiency symptoms,
Sulphur deficiency symptoms resemble nitrogen deficiency symptoms because both are related to protein and chlorophyll deficiency.
Fertilizers: gypsum and elemental sulphur, both of which are also used to lower soil pH; ammonium sulphate, superphosphate potassium sulphate (VI).
Iron
Occurrence: In igneous rocks, iron occurs in the Fe2+ form. The iron in water-logged soils tends to remain in this form and contributes to the bluish-grey colours that indicate wetness. Much of the iron in well drained soils is in the Fe3+ form and is associated with humus and mineral particles.
Availability: Plants absorb iron in the form of Fe2+ and Fe3+.
Use in plants;
Iron is an essential catalyst in the formation of chlorophyll and functions in some of the enzymes of the respiratory system. Iron is needed in larger quantity than all other micronutrients.
Its Deficiency symptoms,
An iron deficiency results in the young leaves being small and pale green or yellow in colour.
Fertilizers: Iron (II) sulphate (FeSO4) which is soluble in water. Application of iron is generally ineffective to calcareous soils.
THE OTHER MICRONUTRIENTS
Availability: Micronutrients are taken in by plants as Bo2–, Co2+, Cu2+, Mn2+, MoO2– and Zn2+. The micronutrients in the soil usually originate from the parent material of the soil. Plant needs of these micronutrients are very small.
Use in plants: Most micronutrients are used as catalysts in plant metabolism:
- Manganese is a catalyst in the formation of chlorophyll and in many redox reactions, e.g. metabolism of nitrogen, iron, copper, zinc and in vitamin C synthesis.
- Boron aids protein synthesis, regulates the K:Ca ratio in plant tissues and is required for the formation of roots and fruits.
- Copper is involved in respiration and in the nitrogen and iron metabolism.
- Molybdenum is essential in the protein synthesis and for the nitrogen fixation by rhizobia on the roots of legumes.
- Zinc catalyses the formation of growth hormones and promotes the synthesis of RNA and chloroplasts. Thus it is essential for normal growth.
- Chlorine seems to be essential in photosynthesis and is required for plant growth.
- Cobalt is essential for nitrogen fixation by rhizobia and hence aids growth of legumes. However, it is not clear whether it is essential for growth of higher plants.
Fertilization: Large amounts of micronutrients are usually toxic to plants. The best method of application is usually foliar spraying.
Preparation of Plant Nutrient Cultures in the Laboratory
Prepare plant nutrient cultures in the laboratory
Nutrient cultures are prepared in the laboratory by using chemicals such as CaSO4, Ca3(PO4)2, MgSO4 and KNO3. These salts are dissolved in water to make cultures containing ions of plant mineral elements. Different cultures lacking some mineral nutrients are made and used to grow plants. The health of plants in various cultures is compared with those grown in a culture with all elements.
Mangaging the Loss of Plant Nutrients from the Soil
Manage the loss of plant nutrients from the soil
Crop plants take up nutrients from the soil continuously. To maintain the soil fertility, the nutrients taken by plants must be replenished (replaced). There are several methods that when combined at least in some aspects can help raise or maintain soil fertility. These are:
Addition of inorganic fertilizers and manure
Inorganic (industrial) fertilizers
Fertilizers are mostly inorganic compounds which contain one or more plant nutrients in a concentrated form. They help to increase or maintain fertility if used carefully with a good background of knowledge. However, if used without proper knowledge or advice by agricultural officers they can be harmful to the soil, crops, animals and humans.
It should also be noted that without reasonable humus content, the soil may have such a low cation exchange capacity that most of the applied fertilizer is leached from the soil instead of being available to plants.
Thus, just adding fertilizer on a field without good cropping system and, advisably, with addition of manure is often a waste of money, time and energy.
Organic fertilizers (manures)
There are different kinds of manures that can be applied to the soil. These include:
- biogas manure - from biogas plants-
- farm yard manure - from wastes of farm animals such as cattle, sheep, goats, poultry, pigs, donkeys, etc;-
- compost manure – from decomposed organic matter; and-
- leguminous green manures, like sunhemp, beans, cowpeas, groundnuts, peas, etc. These young plant materials when ploughed and incorporated into the soil provide organic matter and nitrogen.
Prevention of soil erosion
Most plant nutrients are concentrated on the top soil. If this soil is eroded the nutrients are lost too. This can be stopped by taking soil conservation measures which include mulching terracing/ridging, deep tillage, contour ploughing, strip cropping, planting shelter belts or windbreaks, reforestation, avoiding overgrazing and overstocking, etc.
Crop rotation
This refers to the practice of planting different crops in a field in successive growing seasons. A good crop rotation is that which include leguminous crops (which fix nitrogen in the soil) followed by non-leguminous crops and vice-versa.
Intercropping
Intercropping refers to the act of planting two different crops (preferably legumes with non-legumes) on the same field. Legumes provide nitrogen to non-legumes and the non-legumes help to cover the soil to prevent erosion.
Agroforestry
This refers to mixed cropping of e.g. cereals and usually leguminous trees like Leucaena leucocephala, which provide nitrogen to the field. The trees take up nutrients from the deeper layers of the soil while the cereals take up their nutrients from the top layers. Leguminous trees provide the cereals with the humus when their leaves fall and rot on the soil. They also provide forage for animals, and firewood. Agroforestry is also one of the protections against soil erosion.
Good harvesting practices
Crop remnants harvest should not be burnt down but they must be ploughed and incorporated into the soil. This will help to maintain adequate levels of organic matter in the soil and hence reduce soil erosion. Also by burning crop residues, valuable plant nutrients (N, P and S) are lost and the chance to increase humus level of the soil is reduced.
Prevention of leaching
Leaching refers to loss of plant nutrients from the top to the bottom soil layers, following heavy rains or overirrigation. This can partly be stopped or reduced by maintaining adequate levels of soil organic matter to trap the nutrients and also by avoiding too much irrigation. It can also be stopped by avoiding overcultivation, a fact which makes the soil too loose that the nutrients are easily percolated with the soil solution to the bottom soil layers making these nutrients unavailable to plants.
Following /bush fallow
This refers to leaving the land idle to rest, a fact which allows the land time to regain its lost fertility. However this practice is only possible for farmers with plenty of land. It was an equally good practice in the past when human population was low as compared to vastness of the land at that time. It is not widely practiced today except in areas with low population density and abundant arable land.
Soil conservation
Taking soil conservation measures such as terracing, contour ploughing, mulching, deep tillage, etc. will help maintain soil fertility.
Cultivation of the appropriate crops for the soil
Different crops are suited to different soil types. If you plant crops in a wrong soil you are likely to weaken that soil and destroy its fertility status. But if appropriate crops are planted in the right soil type, chances of maintaining or sustaining the fertility of that soil is also very high.
Good farming practices
Adoption of appropriate soil amendment practice such as liming, acidification, conversion, etc helps to maintain soil fertility. For example, liming is good as it corrects soil acidity but if too much lime (overliming) is applied it results to another problem of setting on soil alkalinity. Likewise, an acid soil, no matter how much beneficial nutrients it contains, is of no use unless its acidity is corrected
Avoidance and/or control of soil pollution
Avoid dependence and overuse of agrochemicals such as pesticides, herbicides and inorganic fertilizers unnecessarily. These chemicals must be used with care and only where agricultural production is impossible without their application. This is because they contribute a great deal to soil pollution and toxification of beneficial soil organisms.
Nutrient balance maintenance
The balance of nutrients in the soil must always be maintained. Plants usually require specific quantities of different nutrient elements. These nutrients must be maintained in the soil by good cropping systems, application of appropriate fertilizers and manure and adopting good soil management practices.
Manures and Fertilizers
Fertilizer refers to any natural/manufactured/synthetic materials that contain at least 5% or more of one or more of the primary plant nutrients/element (N, P or K). Examples of fertilizers include ammonium nitrate, sulphate of ammonia, CAN, NPK, etc.
Manure refers to any fertilizer material, from plant or animal bodies or wastes. Examples of manures include farm yard manure (from domesticated animal wastes), green manure (from young green leguminous plants), and heap and compost manure (from decomposed plants). Manures are sometimes called organic fertilizers.
Preparation of Heap and Pit Compost Manure
Prepare heap and pit compost manure
Steps:
- Dig compost pit;
- Place dry plant materials. Sprinkle enough water;
- The next layer will be composed of green plant materials or any refuse
- Top this with a mixture of animal manure, soil, and ash;
- Repeat steps 2-4 until the pile reaches a height of 1 m;
- Cover the pit with broad-leaved plants;
- Turn the pile every two weeks. The compost is ready after 3-4 months.
The Advantages and Disadvantages of Natural Manures
Explain the advantages and disadvantages of natural manures
Manures have got several advantages and disadvantages. Some of these are explained below:
Advantages
- They add nutrients to the soil and at the same time improve soil physical properties such as soil colour, soil structure and water holding capacity of the soil. A soil with good content of organic matter (supplied by manure) holds water and dissolved nutrients efficiently making them available to crop plants.
- Manures supply humus to soil which, in turn, increases the cation exchange capacity of the soil. Humus accounts for 30–90% of the cation exchange capacity of mineral soils. And because of its high cation exchange capacity, humus helps to store nutrient cations, especially ammonium ions, thus reducing the leaching of these nutrients from the soil.
- Manures improve the proliferation of the soil macro- and microorganisms by supplying the nutrients and conducive conditions needed by these organisms for survival. These organisms play a vital role in soil fertility and plant nutrition by decomposing organic matter which releases nutrients to the
- Manures provide organic matter which acts as the binding materials for soil particles, making them more compact and hence resistant to the impact of rain drops and surface run off of water. Thus, it reduces soil erosion.
- Manures can remain in the soil for a long time and they can provide the nutrients to crops for several growing seasons. Its nutrients are released slowly over a long period of time
- They do not change the soil pH greatly as the inorganic fertilizers do.
- Humus from organic matter is dark in colour and imparts this black colouration to the soil. Black colour absorbs more heat and hence helps to regulate soil temperature.
Disadvantages
- Manures contain and provide little nutrients per unit volume and weight. One has to apply tremendous amounts of manure to meet the requirements of plants.
- Their bulkiness and volume makes it difficult to store, handle or apply in the field. It requires more space to store or transport and more labour to apply manures in the field.
- Some kinds of manures e.g. sludge or industrial and municipal wastes may contain toxic chemicals which can harm humans, animals or soils to which it is applied.
- Manures act very slowly in that they release nutrients to the soil at a very slow rate.
- If the plant materials used to make manure is infested with plant pests or weed seeds; or infected with diseases, there is a risk of spreading them to the farm.
- Manures easily lose nutrients if stored improperly. Under hot conditions, manures produce a lot of heat that leads to loss of136nitrogen through vapourization. Soluble nutrients are easily leached
Types of Synthetic Fertilisers Used in Tanzania
Mention types of synthetic fertilisers used in Tanzania
Farmers in different parts of the country use different fertilizers (depending on soil conditions) to improve soil fertility. Examples of fertilizers used by farmers in Tanzania include:
- Sulphate of ammonia, (NH4)SO4;
- Calcium Ammonium Nitrate, CAN;
- NPK;
- Superphosphates;
- Ammonium chloride, NH4Cl;
- Urea, CO(NH2)2
- Ammonium nitrate, NH4NO3;
- Potassium sulphate, K2SO4
- Potassium chloride, KCl; etc.
The Concept of Fertiliser Grades and Analysis
Explain the concept of fertiliser grades and analysis
A fertilizer is any substance containing plant nutrients that is usually added to soil to supplement the required plant nutrients. Chemical fertilizers may be natural or synthetic. Natural inorganic fertilizers would include materials such as Chile saltpetre (NaNO3), rock phosphate, potassium chloride (KCl), etc. Synthetic fertilizers are manufactured products, such as urea, ammonium sulphate, ammonium phosphate, single superphospahte, etc.
Synthetic fertilizers are available in various grades and analyses. A complete fertilizer has all three primary fertilizer ingredients (N, P and K) as part of its formulation. For example, 14 – 15 – 14 is a complete fertilizer. This fertilizer has 14%N, 15%P and 14%K.
Fertilizers may be classified according to the nutrient elements present. Fertilizers may be:
- Single nutrient fertilizer or straight fertilizers: These are fertilizers containing only one of the primary nutrient elements.
- Double nutrient fertilizer: These contain two primary nutrient elements.
- Complete fertilizers or complex fertilizers: These are materials that contain all three primary elements, N, P and K.
Types of fertilizers
Now let us look at few common fertilizer materials and their grades.
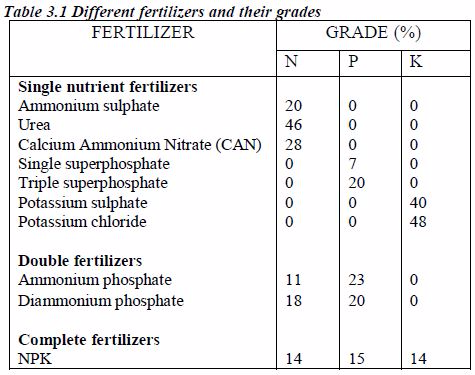
In most of the commercials fertilizers P is expressed as P2O5 and K as K2O. To convert P2O5 to P, multiply the value by 0.437 and to convert K2O to K, multiply the value by 0.830. However, in table 3.1 the nutrient contents are given in elemental form, N, P and K.
The nutrient fertilizer grade indicates that everything in a bag of fertilizer is not plant nutrient. Much of the material in the bag is made up of filler materials. For example, ammonium sulphate contains 20%N. That means, 100 Kg of ammonium sulphate will contain 20 Kg of N and the remaining 80 Kg is sulphate and filler material.
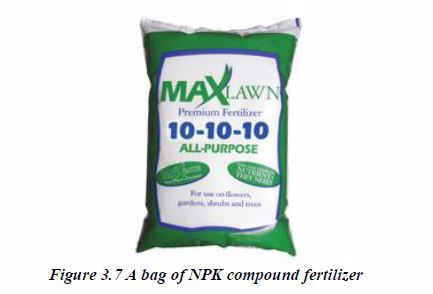
With this background about fertilizer material, we need to calculate the amounts of each nutrient source to meet the fertilizer recommendations. Fertilizer recommendations are expressed in kilograms of nitrogen (N), phosphorus (P) and potassium (K) per hectare. Fertilizers available in the market also contain carrier and filler materials in addition to N, P, or K. Hence, there is need to compute the amount of fertilizer required to supply the recommended rate of nutrients.For example, if 90 Kg of N is recommended for sorghum, we must convert this recommendation to Kg of urea or some other N fertilizer to be applied.
Example 1
A certain soil requires 80 Kg of N per hectare so as to fulfil plantrequirements of nitrogen. Calculate, in kilograms, the quantity ofammonium sulphate fertilizer required to meet this demand.
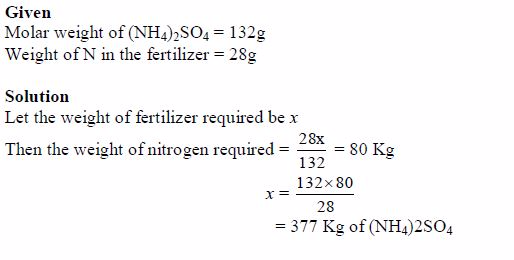
Methods of Fertilizer Application
Identify methods of fertilizer application
Fertilizers can be applied in several ways. The most importantpoint to remember is to apply them at the proper rate, asoverapplication can result in plant damage or death. Follow soilrecommendations or manufacture‟s directions. Some of thecommon fertilizer application methods are as follows:
Broadcasting
Broadcasting refers to spreading the fertilizer uniformly over theentire area before planting and then incorporating it into the soil.Application of high rates of the major nutrients namely, nitrogen,potassium and phosphorus is usually carried out by broadcastingwith a tractor-mounted fertilizer spreader. This allows a specific amount of fertilizer to be spread over the entire under-tree area or in band along the row.
Monitoring of the application rate for the various fertilizers which are used is important. If calibration is not done properly, the quantity applied may be considerably different to the desired rate.
Soon after spreading, the fertilizer should be covered with the surface soil to a depth of 4 to 6 inches or alternatively watered in. On alkaline soils, prompt incorporation into the soil profile is vital for nitrogenous fertilizers as they are readily lost into the atmosphere (volatized) in the form of ammonia if left exposed on the soil surface.
Broadcasting is particularly good:
- In soils whose fertility status is extremely low;
- In closely spaced crops such as rice, wheat, pasture, millet, etc;
- If the fertilizer used is in fine particles (granules or powdered); and
- If the field is properly prepared and is in good tilth.
Fertigation
Fertigation refers to the application of nutrients through an irrigation system. In this method, liquid fertilizers such as liquid ammonia, nitrogen solutions, phosphoric acids and even complete fertilizers are applied to the soil via irrigation water. The nutrients are spread onto the soil in solution and then carried down with the infiltrating water. It provides a simple and effective way to supply nutrients, particularly nitrogen and potassium. Most trickle, jet, spray and sprinkler systems can be used. Do not attempt fertigation using flood or furrow irrigation as the distribution of nutrients will be uneven and leaching will occur.
Fertigation has the advantage of supplying nutrients to the area of greatest root activity that is, the irrigated part of the root zone.Depending on the irrigation system used, applications of fertilizer may need to be split over several irrigations. This also improves the percentage taken up and utilized by the plant. Frequent applications are easy to apply and there is no soil compaction problem as would be the case with broadcasting with a tractor.
Before injecting a nutrient into an irrigation system ensure that the form being used is suitable. Some nutrients are readily soluble in irrigation water while other nutrients must be specially formulated for fertigation. For example, most forms of phosphorus are not suited to fertigation as they tend to precipitate out and block the small orifices of irrigation emitters.
Foliar sprays/applications
This refers to spraying a dilute nutrient solution directly to the plant leaves. Foliar application is used for the correction of trace element deficiencies. It should not be relied upon to supply the total nitrogen, phosphorus, and potassium needs of plants. Commonly required foliar sprays are zinc and manganese. Foliar application has the benefit of rapid response as the nutrients are taken into the plant quickly. Foliar applications of micronutrients, especially iron, may be beneficial when high soil pH conditions make the iron unavailable to plant roots.
Generally, uptake is enhanced by low pH, thus there is benefit in adjusting the pH of the spray solution to slightly acidic.
Often foliar applications are more effective in correcting trace element deficiencies than soil applications. Trace elements are frequently tied up by unfavourable soil condition so that nutrients applied to the soil are bound before the plant can utilize them while foliar application bypasses this problem.Foliar spray can also be used to alleviate deficiency of major elements. This is will provide only temporary relief and should be used only as a quick fix to minimize yield or quality loss due to a sudden deficiency. It is not possible to apply the quantity of the major nutrients that growing plants require by foliar applications. Soil applications should provide over 90% of the quantity of each major nutrient.
Banding
Banding refers to placement of fertilizer 2 to 3 inches to each side and below the seed at planting. This technique is risky as placement too close to the seed or at high rate can cause fertilizer burn and inhibit germination.
This method is ideal:
- where the amount of fertilizer to apply is limited;
- for widely-spaced crops;
- when small labour is required ; and
- in seedbed preparation.
Side-dressing
Side-dressing refers to placing the fertilizer beside the row during the growing season. This technique is usually used to apply additional nitrogen during the growing season and is particularly useful for applying nitrogen on sandy soils.
Top-dressing
Top-dressing is similar to side-dressing except that the fertilizer is applied around the plant. Care must be taken not to apply the fertilizer too close to the plant as this can cause fertilizer burn.
Starter Solution Application
Starter solution fertilizers are soluble in water and they are usually high in phosphorus. They are applied as liquid around the plant roots at the time of planting. They are primarily used for vegetable transplants to hasten root development and establishment. Follow manufacturer‟s directions for application rates.
The Advantages and Disadvantages of Artificial Fertilizers as Compared to Natural Manures
Explain the advantages and disadvantages of artificial fertilizers as compared to natural manures
Advantages
- They contain more nutrients per unit volume and weight.
- They are compact and hence easy to transport, store and apply to the field as compared to manures which are bulky in nature.
- They contain specific quantities of plant nutrients per unit volume and weight. So the quantity of a particular nutrient to be applied to the soil can easily be estimated to avoid overapplication. For example the weight of nitrogen in a kilogram of NH4NO3 can easily be established and quantified.
- They dissolve quickly and hence provide nutrients to plants instantly as they are added to the soil.
Disadvantages
- They are used only for one growing season as they are short-lived. Because the uptake of the nutrients in the fertilizer is very high, no or few nutrients would have remained in the soil in the next growing season.
- Some acidic mineral fertilizers such as NH4Cl and (NH4)2SO4 contribute to soil acidity. When these fertilizers are applied to the soil repeatedly, they can make the soil acidic and hence not fit for plant growth.
- Prolonged use of artificial fertilisers may lead to deterioration of the soil structure and poisoning of soil and soil microbes.
- Extensive use of fertilizers may cause contamination of drinking water resources, by especially nitrate fertilizers. Nitrates dissolved in water are not removed by normal purification processes. In the body nitrates may be converted into nitrosamines. These are carcinogenic (cancer-causing) compounds
- If too much nitrogenous fertilizer is used, serious pollution can occur. One kind of pollution is called eutrophication. Excess of the fertilizer applied finally finds its way to water. This encourages fast growth and huge increase in the number of microscopic, aquatic plants called algae, a phenomenon called algal bloom. Proliferation of algae on the water surface blocks sunlight from reaching the plants beneath the water. These plants can not carry out photosynthesis and, therefore, they die. Bacteria and other decomposers feed on these dead plants and increase in number. These decomposers use up all the oxygen dissolved in the water. Without ample supply of oxygen fish and other organisms living in the water die.
- They can scorch (burn) and kill crop plants if not applied under manufacture‟s directions or even harm humans if not properly handled.
- Fertilizers are expensive to purchase and hence not affordable.
Soil Fertility and Productivity
The Concept of Soil Fertility and Soil Productivity
Explain the concept of soil fertility and soil productivity
Soil fertility
Soil fertility is the ability of the soil to supply the essential nutrient elements in adequate amounts, forms, and proportions for maximum plant growth.
There are three types of soil fertility. These are:
- Chemical soil fertility: this is the fertility due to chemical processes that contribute to soil fertility. The chemical soil fertility falls under two categories namely,(i) potential chemical fertility, due to cations in soil solution; and(ii) active chemical fertility, which is due to exchangeable cations adsorbed to the soil colloidal surface or negatively charged plant roots.
- Physical soil fertility: the fertility contributed by soil moisture, texture, structure, temperature, etc.
- Biological soil fertility: this is due to organic matter content, and soil microorganisms.
Soil productivity
Soil productivity is the capacity or ability of a particular soil to sustain plant growth and development. It is measured in terms of yield of a particular crop which is a reflection or consequence of nutrients taken up by plants from the soil. Soil productivity is an interaction of three main factors.
- Soil fertility. This refers to the ability of the soil to supply the essential plant nutrients required for maximum plant growth.
- Plant factors. These includes yield potential, root growth characteristic and genetic make up of a particular crop plant. This means that some crop plants are high-yielding than other plants of the same species and are thus likely to give more crop yields. Also plants with good root development are likely to absorb more nutrients from the soil, grow better and give good yield as compared to plants with poor root development. Genetic make up of a plant also plays a vital role in this respect. For example hybrid maize will always survive harsh146soil and environment conditions than local varieties of maize and, therefore, will give high yields.
- Environment factors. These factors include climatic factors and agronomic practices.Climatic factors – These are factors such as temperature, precipitation (rainfall), radiation, humidity, altitude, etc.Agronomic practices include weed control, pest and disease control, good soil preparation, plant population, etc. Yield can be measured in terms of grain yield, tubers yield, dry matter, height of plants, number of leaves, number and size of fruits, berries, etc.
A soil is considered to productive if:
- It has adequate water retention capacity;
- It is well aerated; and
- It is able to supply adequate amounts of the nutrients to plant.
Water retention capacity is influenced by soil organic matter, soil texture (loam, clay, sand, etc), soil structure, and proportions of macro and micro pores in the soil. Aeration is influenced by soil structure and texture.
Difference between Soil Fertility and Soil Productivity
Differentiate soil fertility form soil productivity
We have learned that soil productivity depends on soil fertility in one way or another. However, plant and climatic factors have their roles play too.
From this point of view, therefore, it is correct to assert that a fertile soil is not necessarily productive simply because soil productivity does not rely singly on the fertility of the soil. It depends on several other factors such as soil moisture, which is determined by climate and even altitude, which influences plant development a great deal. In concise, it should be understood that there are several other factors apart from soil fertility which affects the productivity of the soil. Even soil management practices can affect soil productivity to a large extent. Soil fertility is accounted for by the type and quantity of the nutrient elements present in a particular soil which are available to crop plants.
Soil productivity is a measure of the amount of harvest or yield that can be obtained from a given piece of land under certain agronomic conditions and practices. For example, suppose a farmer grows maize on one acre of plot A and manages to harvest 20 bags of maize. On another one-acre plot, plot B, he harvests only 10 bags of maize. Of the two plots, A is said to be more productive than B. This is one among many means for determining soil productivity.
The Factors which Determine Fertility and Productivity of the Soil
Explain the factors which determine fertility and productivity of the soil
A fertile soil provides all essential plant nutrients in amounts and proportions which are suitable for growth of most plants. Soil fertility depends on a number of factors, namely:
The texture and structure of the soil
This affects water and nutrient storage, and aeration. The soil with a fine texture such as clay contains small airspaces. The movement of air in and out of such a soil is thus minimal. However, these soils have a great capability of holding water and nutrients. Its structure can be corrected by addition of organic matter, such as farm yard manure and compost, and heap manure.
On the other hand, soils having a coarse texture such as sand are quite porous. Sand allows water to pass through it very quickly. It is poor at water retention and nutrient storage. It has wide air spaces and thus well aerated. It can also be improved by adding organic manures.
The depth of the soil profile
The deeper the soil the better the plant root development and the greater the water and nutrient supply potential it has. Shallow soils do not normally allow roots to penetrate deep through the soil. This leads to poor root development and poor plant growth.
The mineral and organic matter content
The chemical composition of the parent material of the soil provides the natural inorganic nutrient supply due to minerals present. A soil formed from the decomposition of limestone will probably contain reasonably high concentrations of Ca2+ ions in their exchangeable sites due to inherent Ca2+ ions derived from limestone. The same case can apply to high contents of nitrogen and phosphorus in humid soils due to high decomposition of organic matter. The soil organic matter helps to cement the soil particles therefore aiding to create a crumbly structure which is ideal for most agronomic practices.
Cation Exchange Capacity (CEC)
This depends very much on the content of the soil and soil pH. A soil well supplied with organic matter has an optimum cation exchange capacity. The cations adsorbed to the soil colloids are easily exchanged with those in the soil solution. Also humus contains humic (organic) acids which can donate protons if the pH is low.
Soil pH
This affects nutrient storage and availability. The availability of N, P, K, S, Ca, Mg, and Mo decreases with increase in soil acidity. Below pH 5 and above pH 7, Al3+ and Fe3+ ions form complexes with soluble phosphates so that the phosphates are no longer available to plants. This is called phosphorus fixation. Below pH 4.8, Al3+ becomes so soluble that it appears in high concentrations in the soil solution which are detrimental to most plants. This aluminium toxicity is a problem in some tropical soils.
Climate
The climate affects water availability, temperature, weathering as well as the physical and chemical properties of the soil. In humid tropical climates the rate of weathering and organic matter decomposition is very high. Soils in wet tropical and equatorial regions are well supplied with water because these regions normally receive sufficient rainfall.
The position of the ground water table
Water table position affects drainage. Normally, the lower the water table the wet is the soil. Such soils have a good content of moisture and soil microorganisms. Usually water rises from the bottom to the upper parts of the soil profile by capillarity action. The presence of a water table can be detected by vegetations growing directly above it, which always remain greenish even during the dry spell.
The Causes of Loss in Soil Fertility
Explain the causes of loss in soil fertility
All factors that contribute to loss of nutrients from the soil cause loss in soil fertility. These factors include:
- Soil erosion: Most plant nutrients are contained in top and sub soil. It is these nutrients that are available to plants for growth and development. When the top player of the soil is removed by erosion, the nutrients in it are lost too. Erosion agents tend to clear and transport the soil from its original site to another site far away. By so doing, the nutrient elements that are contained in this soil are also carried together with the soil. This process then leads to loss of nutrients from the soil and hence loss in soil fertility.
- Leaching: This refers to the flushing of plant nutrients from the top to the lower layers of the soil and beyond the reach of plant150roots. This is caused by heavy rainfall or flood irrigation. The process makes the nutrients unavailable to plants as it washes them for beyond the root zone.
- Monoculture: The cultivation of the same type of crop on a piece of land year after year, leads to soil depletion if manures or fertilizers are not added. Different plants have specific needs for particular mineral compounds. If the same type of crop is grown on as similar field continuously over a number of years, then the soil will become deficient of the minerals taken up by that crop.
- Denitrification: Denitrifying bacteria convert nitrates of the soil to gaseous nitrogen which escapes to the atmosphere, thus depriving the soil of nitrogen. However, this process is counteracted by another type of beneficial bacteria called nitrifying bacteria, which again convert nitrogen of the air back to soil nitrates by a process called nitrification.
- Nutrient uptake by plants: Growing plants absorb nutrients from the soil for growth and development. If the nutrients taken up by plants are not replaced through adding manure or inorganic fertilizers, the soil becomes deficient of these minerals.
- Volatilization: This refers to the conversion of ammonium compounds into ammonia gas. Nitrogenous compounds in the soil are decomposed by heat into ammonia gas. Also when nitrogenous fertilizers are added to highly basic soils, they react with sodium hydroxide in the soil to release ammonia gas which simply escapes to the atmosphere.NH4+(aq) + OH–(aq) → NH3(g) + H2O(l)
- Accumulation of salts: Under normal conditions, rain water washes the mineral salts away, thereby keeping their concentrations in the soil low. However in arid and semi arid151regions the salts accumulate in the soil as the rain falling there is irregular and is insufficient to wash away the salts. This, together with the high evaporation rate and poor drainage, leads to excessive accumulation of salts on or below the soil surface.
- Change in soil pH: Use of acidic fertilizers over a long period of time can make the soil acidic. Change in soil pH affects the activity of soil microorganisms and availability of some plant nutrients. This, in turn, affects the fertility of the soil.
- Burning of vegetation: Burning crop residues and vegetation deprives the soil microorganisms of the organic matter they require for survival and proliferation. This affects microbial activities such as nitrogen fixation and decomposition of organic matter
The act of burning vegetation also exposes the soil to erosion agents such water and wind. The resulting ash also may cause imbalance of nutrients in the soil or make the soil alkaline.
Topic 4
Concept of Pollution
The Concept of Pollution
Explain the concept of pollution
Pollution can also be defined as the introduction of contaminants or pollutants into the natural environment. The environment comprises of living (biotic) and non-living (abiotic) things such as plants, animals, air, land and water.
Contaminants or pollutants are harmful substances introduced into the environment that disturb the balance of nature. Pollution can be in the form of chemical substances or energy such as noise, heat or light.
Human activities play a major role in the pollution of the environment. Humans engage in a myriad of activities such as agriculture, manufacturing, transport, waste disposal, mining etc. All of these activities contribute to environmental pollution in one way or another.
There are three main types of pollution:
- Terrestrial (land) pollution
- Aquatic (water) pollution
- Aerial (air) pollution
Terrestrial Pollution
The Concept of Terrestrial Pollution
Explain the concept of terrestrial pollution
Terrestrial pollution is the degradation or destruction of the earth‟s surface and soil, directly or indirectly, as a result of human activities. The human activities refer to any activities performed by man that lessens the quality and or productivity of the land as an ideal resource for agriculture, forestation, construction, etc.
Human Activities which Cause Terrestrial Pollution
Identify human activities which cause terrestrial pollution
The human activities responsible for this kind of pollution include poor agricultural practices, mining, waste dumping and urban waste disposal. Below are some causes of terrestrial pollution.
Agricultural activities
Because of the ever-increasing human population, demand for food has increased rapidly. Farmers often use fertilizers to increase crop production and pesticides to get rid of pests, fungi and bacteria that destroy the crops or harm animals. The overuse of such agrochemicals results in the contamination and poisoning of the soil. Other causes of soil pollution from agricultural activities include:
- poor methods of irrigation which causes the leaching of cations down the soil surface;
- manure heaped on land, which may leach down the soil; and
- oil spillages that seep into the soil.
Mining activities
During mineral extraction, several land spaces are created beneath the soil surface. The underground holes dug during mining causes the land to sag or subside (caving in). This is nothing but the nature‟s way of filling the spaces left out after mining or extraction activities. This destroys the land and makes it unfit for use.
Mining involves the use of toxic chemicals used for mineral separation. When these chemicals drain into the soil, it gets polluted.
Rain water often leaches harmful substances from the exposed mining waste into the ground. These harmful substances are like heavy metals (e.g. arsenic) and sulphuric acid or chemicals used in processing the ores (e.g. cyanide. These chemicals are another cause of soil contamination.
Deforestation and soil erosion
Deforestation refers to indiscrimate cutting of trees in search of land for agriculture, settlement, mining, industrialization etc. Trees have got a number of advantages which include attracting rainfall, checking soil erosion and breaking strong winds. The act of cutting down trees carelessly leaves the land bare and hence exposes it to agents of erosion.
Soil erosion causes land pollution. The eroded soil loses its nutrients and organic matter, as well as its ability to hold water. As such, soil erosion can render a fertile land as no longer suited for agriculture, or even turn originally fertile lands into barren deserts.
Other than causing soil erosion, deforestation has also been linked to floods which can, in turn, be seen as another cause of land and water pollution.
Sewage disposal
Sewage refers to a waste, in solution or suspension, carried off in sewers or drains with intention of removing it from the community.
In areas where there are no water bodies into which to dump urban sewage directly, it is dumped into sewage pools that are usually dug far away from residential areas to avoid bad smell emitted by the deposited sewage.
The toxins and poisonous chemicals in the sewage gradually seep down into the ground, thus polluting the land and killing beneficial soil microorganisms.
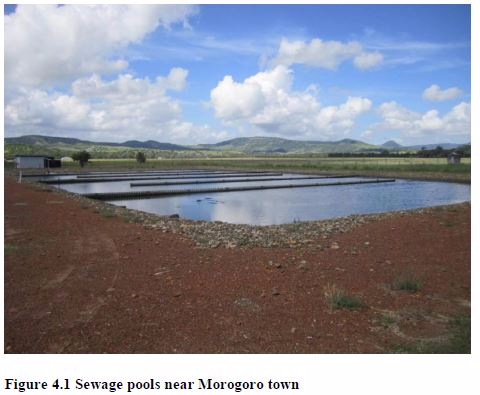
Garbage disposal
Tonnes of garbage are produced each year, especially in urban and industrial areas. Garbage is collected and moved to the dumping sites allocated for that purpose.
The garbage in dumping sites comprises of biodegradable and non-biodegradable wastes. The biodegradable wastes are those that can be broken down easily by the action of microorganisms,e.g. bacteria and fungi. The degradable waste matter may include materials such as rotten foods, kitchen wastes etc.
The non-biodegradable wastes are those that cannot be decomposed by microorganisms and thus remain on the ground for a long time. The non-biodegradable wastes are such as plastic, polythene bags, metal, some clothing and glass.
One of the common causes of land pollution in dumping sites is the contamination of the soil with toxic and even hazardous substances. Needless to say, the ability of this soil to support life is significantly affected.
Transfer of toxic wastes
Industries in developed countries produce much toxic wastes, including the deadly, reactive nuclear wastes. However, these countries have very strict laws which prohibit the dumping of such toxic wastes in their countries. The easiest and cheapest alternative is to dump them in the developing third-world countries. This is because of the greedy and selfish leaders in poor countries are easily that agree to sign contracts to allow the disposal of such dangerous wastes on their lands for their own economic gains.
Hazards Caused by Terrestrial Pollution
Identify hazards caused by terrestrial pollution
The extent of terrestrial pollution is often overlooked because its effects are not well evident to most people. However, land pollution has got a number of negative effects to soil, soil organisms, man, plants and animals. The following are some effects of terrestrial pollution:
- Wastes dumped carelessly can endanger the health of man as well as other organisms. Broken glass, metal and other sharp objects may pierce one‟s skin and introduce disease germs into the body. Empty cans, glass and plastic containers are potential breeding grounds for mosquitoes which spread malaria and other diseases. Rotten organic matter may harbour many disease germs and they also produce noxious smell when they rot. The rotten wastes also attract flies which transmit a number of enteric diseases like dysentery, cholera, diarrhoea, etc.
- Land pollution causes chemical contamination to the ecosystem. This occurs when the chemicals in the waste matter poison the soil. Then plants growing on the poisoned soil, animals that eat these plants and even humans are all affected by these chemicals. This process is called biomagnifications and is a serious threat to the ecology. It can lead to the loss of some types of plants and animal life as well as create long-term health problems such as cancer and other deformities in humans. Radiation from nuclear wastes causes healthy problems such as cancers and other deformities.
- Piles of waste in urban areas keep growing due to increase in waste. When this waste is burned it produces a lot of smoke that leads to air pollution.
- Soil erosion (as a form of land pollution) leads to loss of land for agriculture, settlement, forest cover, fodder patches for grazing, etc.
- Land pollution leads to loss of ecosystem and hence directly or indirectly cause change in climatic patterns.
- Deforestation causes imbalance in the rain cycle. A disturbed rain cycle affects a lot of factors such as reduction in the green cover. Plants help absorb excess carbon dioxide from the air and release oxygen to the atmosphere. This process helps to balance the atmosphere. Without vegetation cover, excessive accumulation of carbon dioxide in the atmosphere causesconcerns like global warming, the greenhouse effect, irregular rainfall, and floods among other imbalances.
- Land pollution damages terrestrial life, especially plants. This greatly affects wildlife and other animal species which are forced to move further away and adapt to new regions or die trying to adjust.
- Heaps of different wastes from mining activities make the environment unsightly and ugly.
- Terrestrial pollution is a big problem in urban areas where waste production outweighs waste disposal. In such areas you find poor and blocked sewage system, effluent from domestic toilets flowing on the streets and roads, and dirty water carelessly poured on the ground. This makes life in urban areas uncomfortable and a mere nuisance.
Different Methods of Preventing Terrestrial Pollution
Suggest different methods of preventing terrestrial pollution
The following are some measures that can be taken to prevent and control land pollution:
Recycling and reuse
Recycling is the processing of changing used materials into usable raw materials instead of discarding them as wastes altogether. Scrap metals, plastic bottles and glass should be recycled instead of being dumped into the environment. Packaging materials such as plastic bags, beverage and water bottles can be recycled or re-used for packaging or carrying goods.
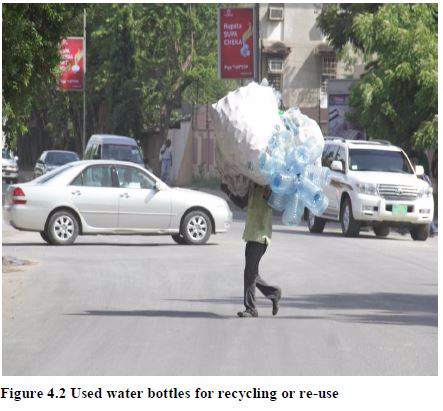
Using biodegradable materials
Emphasis should be put on manufacturing and using materials that can easily be broken down (biodegradable). For example, paper bags should be manufactured and used instead of plastic (polythene) bags, which are non-biodegradable. Biodegradable plastics have been developed and are used. Some biodegradable plastics include
- biopolymers such as those used in making surgical sutures;
- photodegradable plastics, which break down upon exposure to light: and
- soluble plastics which can be broken down by water
Proper disposal of wastes
Urban waste should only be dumped in allocated dumping sites. These sites must be far away from residential areas to avoid the risk of spreading diseases, pest infestation and a bad smell. The sewage should be properly treated before being drained into dumping pools. Some of the methods that can be used to curb urban waste problem include incineration and recycling. Paraffin should be poured onto the sewage pools to suffocate and kill mosquito larvae and hence prevent the spread of malaria to residents living in the vicinity of the dumping pools.
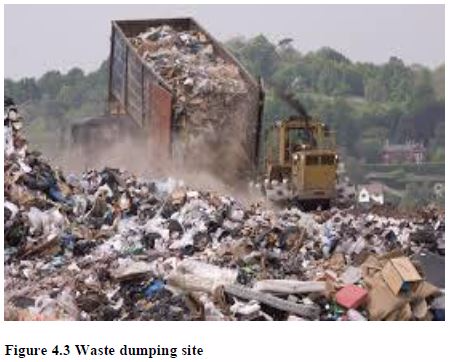
Reducing the use of agrochemicals
Farmers should be advised and encouraged to avoid dependency on agricultural chemicals (fertilizers, herbicides pesticides, etc). All of these chemicals pollute the soil and affect soil microorganisms a great deal. Farmers should use organic manures and chemicals in their agricultural operations. These do not pollute the land or affect crops and animals as compared to artificial chemicals. They help to improve soil structure and hence prevent soil erosion.
Making and enacting environmental laws and policies
The government should make and implement laws and regulations to prevent and control terrestrial pollution. Likewise, local government authorities should make by-laws aiming at curbing the problem of environmental pollution. The laws must clearly state guidelines and procedures to be followed by everybody regarding environment sanitation. These may include:
- discharge and treatment of sewage;
- disposal of lethal nuclear wastes;
- use of agrochemicals in agricultural production;
- use of plastic and related materials;
- disposal of toxic chemicals and solid wastes from industries; and
- careless littering of the environment by irresponsible people.
Creating public awareness
The general public should be educated about the importance of keeping the environment clean and the benefit of living in a clean environment. This knowledge can be conveyed through meetings or via mass media such as television, radio and newspapers. It may also be conveyed via announcements, posters and other social media.
Tags:
Related Posts
PHYSICAL CHEMISTRY NGAIZA FULL NOTES PDF
May 10, 20200PHYSICS FORM FOUR
May 02, 20200PHYSICS FORM THREE
May 01, 20200
No comments